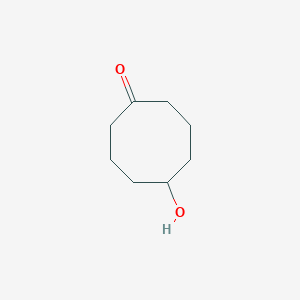
5-Hydroxycyclooctanone
Overview
Description
5-Hydroxycyclooctanone is a chemical compound with the molecular formula C8H14O2 . It has a molecular weight of 142.2 and is typically found in a powder form .
Synthesis Analysis
The synthesis of this compound can be achieved with an alcohol and hydrochloric acid . Another method involves the reaction of this compound with O-methylhydroxylammonium chloride and sodium acetate in methanol at room temperature .Molecular Structure Analysis
The InChI code for this compound is 1S/C8H14O2/c9-7-3-1-4-8(10)6-2-5-7/h7,9H,1-6H2 . This indicates that the molecule consists of 8 carbon atoms, 14 hydrogen atoms, and 2 oxygen atoms.Chemical Reactions Analysis
One notable chemical reaction involving this compound is the transannular 1,5-hydride shift . This reaction has been analyzed by quantum chemical calculations and involves a degenerate transannular 1,5-hydride shift .Physical and Chemical Properties Analysis
This compound is a powder at room temperature . It has a melting point of 99-100 degrees Celsius .Scientific Research Applications
Chemical Properties and Synthesis
Protection and Reactivity
5-Hydroxycyclooctanone exhibits interesting reactivity due to its medium-ring hydroxy ketone structure. It predominately exists as the transannular hemiacetal and can be protected with an alcohol and hydrochloric acid. This process involves a 1,5-hydride shift, indicating its unique reactivity and potential for further chemical transformations (Choudhary Mohr & Rademacher, 2008).
Transannular Hydride Shift
Further analysis of this compound revealed a transannular 1,5-hydride shift mechanism, confirmed through 1H NMR measurements and quantum chemical calculations. This study highlights its potential in synthetic organic chemistry, demonstrating the molecule's intriguing behavior under both acidic and basic conditions (Rademacher & Choudhary Mohr, 2007).
Biological Implications
Stability and Role in DNA
While not directly related to this compound, the study of 5-Hydroxymethylcytosine, a derivative of 5-methylcytosine in DNA, has profound implications in understanding DNA methylation and potential biological roles. Such studies provide a framework for investigating similar molecules like this compound in biological systems, examining their stability, and exploring their roles in health and disease (Bachman et al., 2014).
Epigenetic Modifications
Research into 5-Hydroxymethylcytosine also sheds light on the complexity of epigenetic modifications, where molecules similar to this compound might play a role. Understanding these modifications is crucial for grasping the full scope of genetic regulation and expression in various cell types, including embryonic stem cells (Pastor et al., 2011).
Mechanism of Action
Target of Action
The primary target of 5-Hydroxycyclooctanone is the methylene hydrogen atoms present in its structure . The compound interacts with these atoms under certain conditions, leading to a change in their state .
Mode of Action
This compound undergoes a process known as a transannular 1,5-hydride shift . This involves the exchange of eight out of twelve methylene hydrogen atoms for deuterium atoms under acidic and basic conditions . The reaction takes place via a tight transition state with a six-membered ring . The activation barrier for this process is lowest in the presence of a base .
Biochemical Pathways
The primary biochemical pathway affected by this compound is the transannular 1,5-hydride shift . This process results in the exchange of hydrogen atoms for deuterium atoms, altering the structure of the compound
Pharmacokinetics
The compound’s reactivity under acidic and basic conditions suggests that its bioavailability may be influenced by the ph of its environment .
Result of Action
The primary result of this compound’s action is the exchange of methylene hydrogen atoms for deuterium atoms . This alters the structure of the compound, potentially influencing its biochemical activity .
Action Environment
The action of this compound is influenced by the acidity or basicity of its environment . The compound’s reactivity is highest under basic conditions, suggesting that its efficacy and stability may be affected by pH .
Safety and Hazards
Properties
IUPAC Name |
5-hydroxycyclooctan-1-one | |
---|---|---|
Source | PubChem | |
URL | https://pubchem.ncbi.nlm.nih.gov | |
Description | Data deposited in or computed by PubChem | |
InChI |
InChI=1S/C8H14O2/c9-7-3-1-4-8(10)6-2-5-7/h7,9H,1-6H2 | |
Source | PubChem | |
URL | https://pubchem.ncbi.nlm.nih.gov | |
Description | Data deposited in or computed by PubChem | |
InChI Key |
HUNLAYAAWSTPQY-UHFFFAOYSA-N | |
Source | PubChem | |
URL | https://pubchem.ncbi.nlm.nih.gov | |
Description | Data deposited in or computed by PubChem | |
Canonical SMILES |
C1CC(CCCC(=O)C1)O | |
Source | PubChem | |
URL | https://pubchem.ncbi.nlm.nih.gov | |
Description | Data deposited in or computed by PubChem | |
Molecular Formula |
C8H14O2 | |
Source | PubChem | |
URL | https://pubchem.ncbi.nlm.nih.gov | |
Description | Data deposited in or computed by PubChem | |
DSSTOX Substance ID |
DTXSID30298711 | |
Record name | 5-hydroxycyclooctanone | |
Source | EPA DSSTox | |
URL | https://comptox.epa.gov/dashboard/DTXSID30298711 | |
Description | DSSTox provides a high quality public chemistry resource for supporting improved predictive toxicology. | |
Molecular Weight |
142.20 g/mol | |
Source | PubChem | |
URL | https://pubchem.ncbi.nlm.nih.gov | |
Description | Data deposited in or computed by PubChem | |
CAS No. |
61755-97-3 | |
Record name | NSC125566 | |
Source | DTP/NCI | |
URL | https://dtp.cancer.gov/dtpstandard/servlet/dwindex?searchtype=NSC&outputformat=html&searchlist=125566 | |
Description | The NCI Development Therapeutics Program (DTP) provides services and resources to the academic and private-sector research communities worldwide to facilitate the discovery and development of new cancer therapeutic agents. | |
Explanation | Unless otherwise indicated, all text within NCI products is free of copyright and may be reused without our permission. Credit the National Cancer Institute as the source. | |
Record name | 5-hydroxycyclooctanone | |
Source | EPA DSSTox | |
URL | https://comptox.epa.gov/dashboard/DTXSID30298711 | |
Description | DSSTox provides a high quality public chemistry resource for supporting improved predictive toxicology. | |
Record name | 5-hydroxycyclooctan-1-one | |
Source | European Chemicals Agency (ECHA) | |
URL | https://echa.europa.eu/information-on-chemicals | |
Description | The European Chemicals Agency (ECHA) is an agency of the European Union which is the driving force among regulatory authorities in implementing the EU's groundbreaking chemicals legislation for the benefit of human health and the environment as well as for innovation and competitiveness. | |
Explanation | Use of the information, documents and data from the ECHA website is subject to the terms and conditions of this Legal Notice, and subject to other binding limitations provided for under applicable law, the information, documents and data made available on the ECHA website may be reproduced, distributed and/or used, totally or in part, for non-commercial purposes provided that ECHA is acknowledged as the source: "Source: European Chemicals Agency, http://echa.europa.eu/". Such acknowledgement must be included in each copy of the material. ECHA permits and encourages organisations and individuals to create links to the ECHA website under the following cumulative conditions: Links can only be made to webpages that provide a link to the Legal Notice page. | |
Retrosynthesis Analysis
AI-Powered Synthesis Planning: Our tool employs the Template_relevance Pistachio, Template_relevance Bkms_metabolic, Template_relevance Pistachio_ringbreaker, Template_relevance Reaxys, Template_relevance Reaxys_biocatalysis model, leveraging a vast database of chemical reactions to predict feasible synthetic routes.
One-Step Synthesis Focus: Specifically designed for one-step synthesis, it provides concise and direct routes for your target compounds, streamlining the synthesis process.
Accurate Predictions: Utilizing the extensive PISTACHIO, BKMS_METABOLIC, PISTACHIO_RINGBREAKER, REAXYS, REAXYS_BIOCATALYSIS database, our tool offers high-accuracy predictions, reflecting the latest in chemical research and data.
Strategy Settings
Precursor scoring | Relevance Heuristic |
---|---|
Min. plausibility | 0.01 |
Model | Template_relevance |
Template Set | Pistachio/Bkms_metabolic/Pistachio_ringbreaker/Reaxys/Reaxys_biocatalysis |
Top-N result to add to graph | 6 |
Feasible Synthetic Routes
Disclaimer and Information on In-Vitro Research Products
Please be aware that all articles and product information presented on BenchChem are intended solely for informational purposes. The products available for purchase on BenchChem are specifically designed for in-vitro studies, which are conducted outside of living organisms. In-vitro studies, derived from the Latin term "in glass," involve experiments performed in controlled laboratory settings using cells or tissues. It is important to note that these products are not categorized as medicines or drugs, and they have not received approval from the FDA for the prevention, treatment, or cure of any medical condition, ailment, or disease. We must emphasize that any form of bodily introduction of these products into humans or animals is strictly prohibited by law. It is essential to adhere to these guidelines to ensure compliance with legal and ethical standards in research and experimentation.