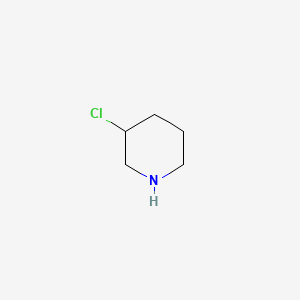
3-Chloropiperidine
Overview
Description
3-Chloropiperidine is a chemical compound with the formula C5H10ClN . It is an isomer of chloropyridine . It is mainly used as a building block in organic synthesis .
Synthesis Analysis
The synthesis of novel secondary this compound analogues incorporates a new procedure to monochlorinate unsaturated primary amines utilizing N-chlorosuccinimide . The temperature is carefully monitored to prevent dichlorination . Furthermore, highly strained bicyclic aziridines are successfully isolated by treating the secondary 3-chloropiperidines with a sufficient amount of base .
Molecular Structure Analysis
This compound is expected to react via a highly electrophilic bicyclic aziridinium ion . This ion is readily attacked by nucleophiles .
Chemical Reactions Analysis
3-Chloropiperidines are potent chemotherapeutics, alkylating the DNA through the formation of bicyclic aziridinium ions . The secondary this compound, as well as the isolated bicyclic aziridine, proved to be more effective than their tertiary counterpart .
Physical And Chemical Properties Analysis
This compound is a colorless liquid . It has a molecular formula of C5H10ClN .
Mechanism of Action
Safety and Hazards
Future Directions
properties
IUPAC Name |
3-chloropiperidine | |
---|---|---|
Source | PubChem | |
URL | https://pubchem.ncbi.nlm.nih.gov | |
Description | Data deposited in or computed by PubChem | |
InChI |
InChI=1S/C5H10ClN/c6-5-2-1-3-7-4-5/h5,7H,1-4H2 | |
Source | PubChem | |
URL | https://pubchem.ncbi.nlm.nih.gov | |
Description | Data deposited in or computed by PubChem | |
InChI Key |
YQUBWBSLSNIUBN-UHFFFAOYSA-N | |
Source | PubChem | |
URL | https://pubchem.ncbi.nlm.nih.gov | |
Description | Data deposited in or computed by PubChem | |
Canonical SMILES |
C1CC(CNC1)Cl | |
Source | PubChem | |
URL | https://pubchem.ncbi.nlm.nih.gov | |
Description | Data deposited in or computed by PubChem | |
Molecular Formula |
C5H10ClN | |
Source | PubChem | |
URL | https://pubchem.ncbi.nlm.nih.gov | |
Description | Data deposited in or computed by PubChem | |
DSSTOX Substance ID |
DTXSID20329902 | |
Record name | 3-chloropiperidine | |
Source | EPA DSSTox | |
URL | https://comptox.epa.gov/dashboard/DTXSID20329902 | |
Description | DSSTox provides a high quality public chemistry resource for supporting improved predictive toxicology. | |
Molecular Weight |
119.59 g/mol | |
Source | PubChem | |
URL | https://pubchem.ncbi.nlm.nih.gov | |
Description | Data deposited in or computed by PubChem | |
CAS RN |
50564-59-5 | |
Record name | 3-chloropiperidine | |
Source | EPA DSSTox | |
URL | https://comptox.epa.gov/dashboard/DTXSID20329902 | |
Description | DSSTox provides a high quality public chemistry resource for supporting improved predictive toxicology. | |
Retrosynthesis Analysis
AI-Powered Synthesis Planning: Our tool employs the Template_relevance Pistachio, Template_relevance Bkms_metabolic, Template_relevance Pistachio_ringbreaker, Template_relevance Reaxys, Template_relevance Reaxys_biocatalysis model, leveraging a vast database of chemical reactions to predict feasible synthetic routes.
One-Step Synthesis Focus: Specifically designed for one-step synthesis, it provides concise and direct routes for your target compounds, streamlining the synthesis process.
Accurate Predictions: Utilizing the extensive PISTACHIO, BKMS_METABOLIC, PISTACHIO_RINGBREAKER, REAXYS, REAXYS_BIOCATALYSIS database, our tool offers high-accuracy predictions, reflecting the latest in chemical research and data.
Strategy Settings
Precursor scoring | Relevance Heuristic |
---|---|
Min. plausibility | 0.01 |
Model | Template_relevance |
Template Set | Pistachio/Bkms_metabolic/Pistachio_ringbreaker/Reaxys/Reaxys_biocatalysis |
Top-N result to add to graph | 6 |
Feasible Synthetic Routes
Disclaimer and Information on In-Vitro Research Products
Please be aware that all articles and product information presented on BenchChem are intended solely for informational purposes. The products available for purchase on BenchChem are specifically designed for in-vitro studies, which are conducted outside of living organisms. In-vitro studies, derived from the Latin term "in glass," involve experiments performed in controlled laboratory settings using cells or tissues. It is important to note that these products are not categorized as medicines or drugs, and they have not received approval from the FDA for the prevention, treatment, or cure of any medical condition, ailment, or disease. We must emphasize that any form of bodily introduction of these products into humans or animals is strictly prohibited by law. It is essential to adhere to these guidelines to ensure compliance with legal and ethical standards in research and experimentation.