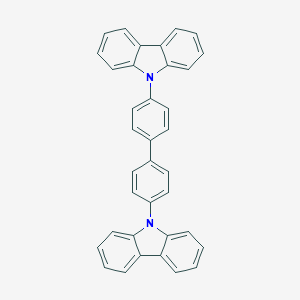
4,4'-Bis(N-carbazolyl)-1,1'-biphenyl
Overview
Description
4,4’-Bis(N-carbazolyl)-1,1’-biphenyl is an organic compound known for its unique properties and applications in various fields, particularly in organic electronics. This compound is characterized by its biphenyl core with two carbazole groups attached at the 4 and 4’ positions. It is widely used as a host material in organic light-emitting diodes (OLEDs) due to its excellent thermal stability, high triplet energy, and good charge-transporting abilities .
Mechanism of Action
Target of Action
The primary target of 4,4’-Bis(N-carbazolyl)-1,1’-biphenyl, also known as 4,4’-Di(9H-carbazol-9-yl)-1,1’-biphenyl, is the electron transport chain in organic light-emitting diodes (OLEDs) . The compound is used as a host material in OLEDs , where it plays a crucial role in transporting electrons and holes .
Mode of Action
The compound interacts with its targets by facilitating the injection and transport of electrons . It has been observed that the introduction of phosphonate substitutes can endow the compound with excellent electron injection/transport ability . As a result, the compound shows an electron-dominated behavior observed in single carrier devices .
Biochemical Pathways
The compound affects the electron transport pathway in OLEDs . The electron transport pathway is crucial for the functioning of OLEDs, as it influences the device’s performance, including its luminous efficiency .
Pharmacokinetics
While the term “pharmacokinetics” is typically used in the context of drug metabolism, in the case of this compound, we can discuss its properties related to its distribution and behavior in the OLEDs. The compound’s highest occupied molecular orbital (HOMO) level is reduced, leading to a large hole injection barrier . This property impacts the compound’s distribution and behavior in the device, influencing its overall performance .
Result of Action
The action of the compound results in a significant increase in the peak luminous efficiency of the OLEDs . Specifically, the peak luminous efficiency significantly increases from 1.7 cd A −1 of CBP to 31.4 cd A −1 of PCBP .
Action Environment
The action, efficacy, and stability of the compound can be influenced by various environmental factors. For instance, the deposition of the compound onto cooled substrates can result in smaller agglomerates, yielding a higher efficiency
Preparation Methods
Synthetic Routes and Reaction Conditions
The synthesis of 4,4’-Bis(N-carbazolyl)-1,1’-biphenyl typically involves the palladium-catalyzed Suzuki-Miyaura cross-coupling reaction. This method allows for the formation of the biphenyl core by coupling two carbazole units with a biphenyl diboronic acid or its ester derivative. The reaction is usually carried out in the presence of a palladium catalyst, such as Pd(PPh3)4, and a base, like potassium carbonate, in a suitable solvent like toluene or dimethylformamide (DMF) under an inert atmosphere .
Industrial Production Methods
In industrial settings, the production of 4,4’-Bis(N-carbazolyl)-1,1’-biphenyl follows similar synthetic routes but on a larger scale. The process involves optimizing reaction conditions to ensure high yield and purity. This includes controlling the temperature, reaction time, and the concentration of reactants. The use of continuous flow reactors and automated systems can enhance the efficiency and scalability of the production process .
Chemical Reactions Analysis
Types of Reactions
4,4’-Bis(N-carbazolyl)-1,1’-biphenyl undergoes various chemical reactions, including:
Common Reagents and Conditions
Reduction: Reducing agents such as lithium aluminum hydride (LiAlH4) and sodium borohydride (NaBH4) are typically used.
Substitution: Electrophilic reagents like alkyl halides and acyl chlorides are used in the presence of a base to facilitate substitution reactions.
Major Products Formed
Oxidation: Dicarbazolyl derivatives and other oxidized products.
Reduction: Carbazole anions and reduced biphenyl derivatives.
Substitution: Functionalized carbazole derivatives with various substituents.
Scientific Research Applications
4,4’-Bis(N-carbazolyl)-1,1’-biphenyl has a wide range of applications in scientific research:
Organic Electronics: It is extensively used as a host material in OLEDs due to its high triplet energy and excellent charge-transporting properties.
Photovoltaics: The compound is used in organic solar cells to enhance charge transport and improve efficiency.
Sensors: Its unique electronic properties make it suitable for use in chemical sensors and biosensors.
Electrochromic Devices: The compound is employed in electrochromic devices for its ability to undergo reversible redox reactions.
Comparison with Similar Compounds
Similar Compounds
4,4’-Bis(N-carbazolyl)-2,2’-biphenyl: Similar structure but with carbazole groups at the 2 and 2’ positions.
1,3-Bis(N-carbazolyl)benzene: Another carbazole-based compound used in OLEDs.
Uniqueness
4,4’-Bis(N-carbazolyl)-1,1’-biphenyl is unique due to its specific positioning of carbazole groups, which provides a balance of electron and hole transport properties. This makes it particularly effective as a host material in OLEDs, offering high thermal stability and efficient charge transport .
Properties
IUPAC Name |
9-[4-(4-carbazol-9-ylphenyl)phenyl]carbazole | |
---|---|---|
Source | PubChem | |
URL | https://pubchem.ncbi.nlm.nih.gov | |
Description | Data deposited in or computed by PubChem | |
InChI |
InChI=1S/C36H24N2/c1-5-13-33-29(9-1)30-10-2-6-14-34(30)37(33)27-21-17-25(18-22-27)26-19-23-28(24-20-26)38-35-15-7-3-11-31(35)32-12-4-8-16-36(32)38/h1-24H | |
Source | PubChem | |
URL | https://pubchem.ncbi.nlm.nih.gov | |
Description | Data deposited in or computed by PubChem | |
InChI Key |
VFUDMQLBKNMONU-UHFFFAOYSA-N | |
Source | PubChem | |
URL | https://pubchem.ncbi.nlm.nih.gov | |
Description | Data deposited in or computed by PubChem | |
Canonical SMILES |
C1=CC=C2C(=C1)C3=CC=CC=C3N2C4=CC=C(C=C4)C5=CC=C(C=C5)N6C7=CC=CC=C7C8=CC=CC=C86 | |
Source | PubChem | |
URL | https://pubchem.ncbi.nlm.nih.gov | |
Description | Data deposited in or computed by PubChem | |
Molecular Formula |
C36H24N2 | |
Source | PubChem | |
URL | https://pubchem.ncbi.nlm.nih.gov | |
Description | Data deposited in or computed by PubChem | |
DSSTOX Substance ID |
DTXSID30460032 | |
Record name | 9,9'-([1,1'-Biphenyl]-4,4'-diyl)di(9H-carbazole) | |
Source | EPA DSSTox | |
URL | https://comptox.epa.gov/dashboard/DTXSID30460032 | |
Description | DSSTox provides a high quality public chemistry resource for supporting improved predictive toxicology. | |
Molecular Weight |
484.6 g/mol | |
Source | PubChem | |
URL | https://pubchem.ncbi.nlm.nih.gov | |
Description | Data deposited in or computed by PubChem | |
CAS No. |
58328-31-7 | |
Record name | 9,9'-([1,1'-Biphenyl]-4,4'-diyl)di(9H-carbazole) | |
Source | EPA DSSTox | |
URL | https://comptox.epa.gov/dashboard/DTXSID30460032 | |
Description | DSSTox provides a high quality public chemistry resource for supporting improved predictive toxicology. | |
Record name | 4,4'-Bis(9H-carbazol-9-yl)biphenyl | |
Source | European Chemicals Agency (ECHA) | |
URL | https://echa.europa.eu/information-on-chemicals | |
Description | The European Chemicals Agency (ECHA) is an agency of the European Union which is the driving force among regulatory authorities in implementing the EU's groundbreaking chemicals legislation for the benefit of human health and the environment as well as for innovation and competitiveness. | |
Explanation | Use of the information, documents and data from the ECHA website is subject to the terms and conditions of this Legal Notice, and subject to other binding limitations provided for under applicable law, the information, documents and data made available on the ECHA website may be reproduced, distributed and/or used, totally or in part, for non-commercial purposes provided that ECHA is acknowledged as the source: "Source: European Chemicals Agency, http://echa.europa.eu/". Such acknowledgement must be included in each copy of the material. ECHA permits and encourages organisations and individuals to create links to the ECHA website under the following cumulative conditions: Links can only be made to webpages that provide a link to the Legal Notice page. | |
Synthesis routes and methods I
Procedure details
Synthesis routes and methods II
Procedure details
Synthesis routes and methods III
Procedure details
Synthesis routes and methods IV
Procedure details
Retrosynthesis Analysis
AI-Powered Synthesis Planning: Our tool employs the Template_relevance Pistachio, Template_relevance Bkms_metabolic, Template_relevance Pistachio_ringbreaker, Template_relevance Reaxys, Template_relevance Reaxys_biocatalysis model, leveraging a vast database of chemical reactions to predict feasible synthetic routes.
One-Step Synthesis Focus: Specifically designed for one-step synthesis, it provides concise and direct routes for your target compounds, streamlining the synthesis process.
Accurate Predictions: Utilizing the extensive PISTACHIO, BKMS_METABOLIC, PISTACHIO_RINGBREAKER, REAXYS, REAXYS_BIOCATALYSIS database, our tool offers high-accuracy predictions, reflecting the latest in chemical research and data.
Strategy Settings
Precursor scoring | Relevance Heuristic |
---|---|
Min. plausibility | 0.01 |
Model | Template_relevance |
Template Set | Pistachio/Bkms_metabolic/Pistachio_ringbreaker/Reaxys/Reaxys_biocatalysis |
Top-N result to add to graph | 6 |
Feasible Synthetic Routes
Q1: What is the molecular formula and weight of CBP?
A1: CBP has a molecular formula of C36H24N2 and a molecular weight of 492.60 g/mol.
Q2: What is the glass transition temperature (Tg) of CBP?
A2: The glass transition temperature of CBP is relatively high, measured at 151 °C. [] This thermal stability makes CBP suitable for use in OLED devices that can reach high operating temperatures.
Q3: How does the molecular structure of CBP influence its film formation and morphology?
A3: Molecular dynamics simulations of CBP in chloroform solution reveal that during solvent evaporation, solute aggregation initiates at the solution-vapor interface, leading to non-homogeneous solvent distribution within the film. [] This non-uniformity can induce preferential alignment of host molecules and likely results in some solvent remaining trapped within the film.
Q4: Can the orientation of CBP molecules within a thin film be controlled?
A4: Yes, studies using vibrational sum frequency generation (VSFG) spectroscopy show that the orientation of CBP molecules at interfaces can be influenced by the substrate. [] For instance, CBP molecules tend to orient more vertically (edge-on) at a buried CaF2 interface compared to a free air interface, likely due to intermolecular π-π stacking interactions.
Q5: Does the deposition temperature affect the orientation of CBP molecules in thin films?
A5: Yes, studies have shown that CBP molecules adopt random orientations in films fabricated at higher temperatures (350 K). [] This random orientation leads to lower hole mobility in the film. Conversely, lower deposition temperatures can promote a more horizontal orientation of CBP molecules.
Q6: What is the primary role of CBP in OLEDs?
A6: CBP is commonly employed as a host material in the emissive layer of OLEDs, particularly for phosphorescent and thermally activated delayed fluorescence (TADF) devices. [, ]
Q7: Why is CBP considered a suitable host material for phosphorescent OLEDs?
A7: CBP possesses suitable energy levels and triplet energy that allow for efficient energy transfer to phosphorescent dopants, resulting in efficient light emission. [] This property has been demonstrated in devices utilizing red phosphorescent iridium(III) complexes doped into CBP.
Q8: Can CBP be used in solution-processed OLEDs?
A9: Yes, CBP is soluble in common organic solvents, enabling its use in solution-processing techniques for OLED fabrication. [, ] This is advantageous for large-area and flexible OLED applications.
Q9: How does the deposition rate of CBP affect the performance of OLEDs?
A10: Research indicates that while high deposition rates of CBP (up to 50 Å/s) do not lead to gas-phase nucleation, they can cause increased efficiency roll-off at high currents due to enhanced triplet-polaron annihilation. [] This effect is attributed to stress accumulation within the CBP film, generating defect states that act as recombination sites.
Q10: How does CBP compare to other host materials in terms of its impact on the efficiency roll-off in TADF-based OLEDs?
A12: Studies have shown that the molecular orientation of the host material can significantly influence the efficiency roll-off characteristics in TADF OLEDs. For instance, randomly oriented CBP molecules, achieved by fabrication at high temperatures, led to a 30% suppression of efficiency roll-off at high current densities compared to more ordered CBP films. []
Q11: What is the role of CBP in hybrid charge transfer exciton (HCTE) confinement?
A14: Research shows that in organic-inorganic quantum wells (QWs) utilizing CBP, the confinement of HCTEs is less efficient compared to QWs using tetraphenyldibenzoperiflanthene (DBP). [] This difference is attributed to the lower energy of the highest occupied molecular orbital (HOMO) level in CBP compared to DBP.
Q12: How do metal nanoparticles influence the performance of CBP-based OLEDs?
A15: Studies on blue micro-OLEDs incorporating aluminum nanoparticle arrays on the anode revealed a suppression of exciplex emission at the NPB/CBP interface. [] Additionally, the localized surface plasmon resonance (LSPR) of the nanoparticles enhanced CBP emission due to energy coupling, ultimately improving device efficiency.
Q13: How does the presence of ZnCl2 affect the photoluminescence of CBP derivatives?
A16: Interestingly, certain CBP derivatives exhibit a strong and selective spectral response to ZnCl2. [] This property makes them potential candidates for developing optical sensors for this specific analyte.
Q14: How does CBP affect the stability of OLED devices?
A17: The high glass transition temperature of CBP contributes to improved thermal stability of OLED devices, allowing for operation at higher temperatures without significant degradation. []
Q15: How does CBP influence the charge balance in OLEDs?
A19: Studies on green phosphorescent OLEDs employing CBP in mixed host emission layers (MH-EMLs) have demonstrated improved charge balance compared to single-host devices. [] This is attributed to the confinement of holes in the EML due to the deep HOMO level of CBP, facilitating more efficient exciton formation and a wider recombination zone.
Q16: How is computational chemistry used to study CBP and its derivatives?
A20: Computational methods like density functional theory (DFT) are employed to investigate the electronic structure, optical properties, and charge transport characteristics of CBP and its derivatives. [] These calculations aid in understanding the relationship between molecular structure and material properties, guiding the design of new and improved OLED materials.
Q17: Are there specific force field parameters tailored for simulating CBP in solid-state simulations?
A21: Yes, researchers have developed united atom force field parameters specifically optimized for simulating CBP and other OLED materials in the solid phase. [] This force field accurately reproduces experimental crystal structures and melting temperatures, making it valuable for studying the morphology of emissive layers in OLED devices.
Disclaimer and Information on In-Vitro Research Products
Please be aware that all articles and product information presented on BenchChem are intended solely for informational purposes. The products available for purchase on BenchChem are specifically designed for in-vitro studies, which are conducted outside of living organisms. In-vitro studies, derived from the Latin term "in glass," involve experiments performed in controlled laboratory settings using cells or tissues. It is important to note that these products are not categorized as medicines or drugs, and they have not received approval from the FDA for the prevention, treatment, or cure of any medical condition, ailment, or disease. We must emphasize that any form of bodily introduction of these products into humans or animals is strictly prohibited by law. It is essential to adhere to these guidelines to ensure compliance with legal and ethical standards in research and experimentation.