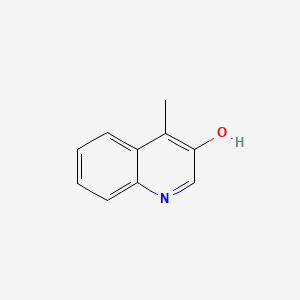
4-Methylquinolin-3-ol
Overview
Description
4-Methylquinolin-3-ol is an organic compound with the chemical formula C({10})H({9})NO. It is a derivative of quinoline, characterized by a methyl group at the fourth position and a hydroxyl group at the third position on the quinoline ring. This compound is known for its applications in various fields, including dye manufacturing, pharmaceuticals, and chemical research.
Mechanism of Action
Target of Action
4-Methylquinolin-3-ol is a derivative of quinoline . Quinoline derivatives are known to have a wide range of pharmacological properties and are used in various fields such as medicine, food, catalysts, dyes, materials, refineries, electronics, etc . .
Mode of Action
Quinoline derivatives are known to inhibit dna synthesis by promoting cleavage of bacterial dna gyrase and type iv topoisomerase, ultimately leading to rapid bacterial death
Biochemical Pathways
Quinoline derivatives are known to have a wide range of biological activities, including antimalarial, antimicrobial, antimycobacterial, antidepressant, anticonvulsant, antiviral, anticancer, antihypertensive, and anti-inflammatory effects . These activities suggest that quinoline derivatives likely interact with multiple biochemical pathways.
Pharmacokinetics
It is known that the compound has high gastrointestinal absorption and is a permeant of the blood-brain barrier . It is also known to inhibit CYP1A2, an enzyme involved in drug metabolism .
Action Environment
It is known that the compound is soluble in water , which could potentially influence its distribution and action in aqueous environments within the body.
Biochemical Analysis
Biochemical Properties
Quinoline derivatives are known to interact with various enzymes and proteins
Cellular Effects
Quinoline derivatives have been found to exhibit antimicrobial, antiviral, and anticancer activities . These effects are likely due to the influence of these compounds on cell signaling pathways, gene expression, and cellular metabolism .
Molecular Mechanism
It is likely that it exerts its effects at the molecular level through binding interactions with biomolecules, enzyme inhibition or activation, and changes in gene expression .
Metabolic Pathways
Quinoline derivatives are known to interact with various enzymes and cofactors
Transport and Distribution
It is likely that it interacts with various transporters or binding proteins, which could affect its localization or accumulation .
Subcellular Localization
It is possible that it is directed to specific compartments or organelles by targeting signals or post-translational modifications .
Preparation Methods
Synthetic Routes and Reaction Conditions: 4-Methylquinolin-3-ol can be synthesized through several methods. One common approach involves the Skraup synthesis, where aniline derivatives react with glycerol in the presence of an oxidizing agent like nitrobenzene and a catalyst such as sulfuric acid. For this compound, the starting material would be 4-methylaniline.
Industrial Production Methods: In industrial settings, the synthesis of this compound often involves optimized conditions to maximize yield and purity. This includes controlled temperatures, specific catalysts, and purification steps such as recrystallization or distillation to isolate the desired product.
Chemical Reactions Analysis
Types of Reactions: 4-Methylquinolin-3-ol undergoes various chemical reactions, including:
Oxidation: It can be oxidized to form quinoline N-oxide derivatives.
Reduction: Reduction reactions can convert it into tetrahydroquinoline derivatives.
Substitution: Electrophilic substitution reactions can introduce different functional groups into the quinoline ring.
Common Reagents and Conditions:
Oxidation: Reagents like hydrogen peroxide or peracids under acidic conditions.
Reduction: Catalytic hydrogenation using palladium or platinum catalysts.
Substitution: Halogenation using reagents like bromine or chlorine in the presence of a Lewis acid catalyst.
Major Products:
Oxidation: Quinoline N-oxides.
Reduction: Tetrahydroquinolines.
Substitution: Halogenated quinolines or other substituted derivatives depending on the reagents used.
Scientific Research Applications
4-Methylquinolin-3-ol has diverse applications in scientific research:
Chemistry: Used as an intermediate in the synthesis of complex organic molecules and dyes.
Biology: Studied for its potential biological activities, including antimicrobial and anticancer properties.
Medicine: Investigated for its role in drug development, particularly in the synthesis of pharmacologically active compounds.
Industry: Utilized in the production of dyes, pigments, and as a sensitizer in photographic films.
Comparison with Similar Compounds
Quinoline: The parent compound, lacking the methyl and hydroxyl groups.
4-Methylquinoline: Similar structure but without the hydroxyl group at the third position.
3-Hydroxyquinoline: Lacks the methyl group at the fourth position.
Uniqueness: 4-Methylquinolin-3-ol is unique due to the presence of both a methyl group and a hydroxyl group, which can significantly influence its chemical reactivity and biological activity compared to its analogs
Properties
IUPAC Name |
4-methylquinolin-3-ol | |
---|---|---|
Source | PubChem | |
URL | https://pubchem.ncbi.nlm.nih.gov | |
Description | Data deposited in or computed by PubChem | |
InChI |
InChI=1S/C10H9NO/c1-7-8-4-2-3-5-9(8)11-6-10(7)12/h2-6,12H,1H3 | |
Source | PubChem | |
URL | https://pubchem.ncbi.nlm.nih.gov | |
Description | Data deposited in or computed by PubChem | |
InChI Key |
UCYWRHDNJCNVNK-UHFFFAOYSA-N | |
Source | PubChem | |
URL | https://pubchem.ncbi.nlm.nih.gov | |
Description | Data deposited in or computed by PubChem | |
Canonical SMILES |
CC1=C(C=NC2=CC=CC=C12)O | |
Source | PubChem | |
URL | https://pubchem.ncbi.nlm.nih.gov | |
Description | Data deposited in or computed by PubChem | |
Molecular Formula |
C10H9NO | |
Source | PubChem | |
URL | https://pubchem.ncbi.nlm.nih.gov | |
Description | Data deposited in or computed by PubChem | |
DSSTOX Substance ID |
DTXSID60211251 | |
Record name | 3-Quinolinol, 4-methyl- | |
Source | EPA DSSTox | |
URL | https://comptox.epa.gov/dashboard/DTXSID60211251 | |
Description | DSSTox provides a high quality public chemistry resource for supporting improved predictive toxicology. | |
Molecular Weight |
159.18 g/mol | |
Source | PubChem | |
URL | https://pubchem.ncbi.nlm.nih.gov | |
Description | Data deposited in or computed by PubChem | |
CAS No. |
6220-93-5 | |
Record name | 3-Quinolinol, 4-methyl- | |
Source | ChemIDplus | |
URL | https://pubchem.ncbi.nlm.nih.gov/substance/?source=chemidplus&sourceid=0006220935 | |
Description | ChemIDplus is a free, web search system that provides access to the structure and nomenclature authority files used for the identification of chemical substances cited in National Library of Medicine (NLM) databases, including the TOXNET system. | |
Record name | 3-Quinolinol, 4-methyl- | |
Source | EPA DSSTox | |
URL | https://comptox.epa.gov/dashboard/DTXSID60211251 | |
Description | DSSTox provides a high quality public chemistry resource for supporting improved predictive toxicology. | |
Retrosynthesis Analysis
AI-Powered Synthesis Planning: Our tool employs the Template_relevance Pistachio, Template_relevance Bkms_metabolic, Template_relevance Pistachio_ringbreaker, Template_relevance Reaxys, Template_relevance Reaxys_biocatalysis model, leveraging a vast database of chemical reactions to predict feasible synthetic routes.
One-Step Synthesis Focus: Specifically designed for one-step synthesis, it provides concise and direct routes for your target compounds, streamlining the synthesis process.
Accurate Predictions: Utilizing the extensive PISTACHIO, BKMS_METABOLIC, PISTACHIO_RINGBREAKER, REAXYS, REAXYS_BIOCATALYSIS database, our tool offers high-accuracy predictions, reflecting the latest in chemical research and data.
Strategy Settings
Precursor scoring | Relevance Heuristic |
---|---|
Min. plausibility | 0.01 |
Model | Template_relevance |
Template Set | Pistachio/Bkms_metabolic/Pistachio_ringbreaker/Reaxys/Reaxys_biocatalysis |
Top-N result to add to graph | 6 |
Feasible Synthetic Routes
Disclaimer and Information on In-Vitro Research Products
Please be aware that all articles and product information presented on BenchChem are intended solely for informational purposes. The products available for purchase on BenchChem are specifically designed for in-vitro studies, which are conducted outside of living organisms. In-vitro studies, derived from the Latin term "in glass," involve experiments performed in controlled laboratory settings using cells or tissues. It is important to note that these products are not categorized as medicines or drugs, and they have not received approval from the FDA for the prevention, treatment, or cure of any medical condition, ailment, or disease. We must emphasize that any form of bodily introduction of these products into humans or animals is strictly prohibited by law. It is essential to adhere to these guidelines to ensure compliance with legal and ethical standards in research and experimentation.