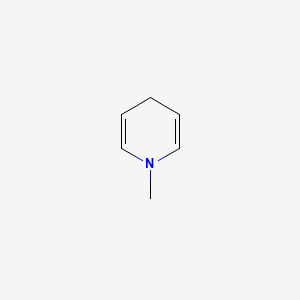
Pyridine, 1,4-dihydro-1-methyl-
- Click on QUICK INQUIRY to receive a quote from our team of experts.
- With the quality product at a COMPETITIVE price, you can focus more on your research.
Overview
Description
Pyridine, 1,4-dihydro-1-methyl- (CAS: Not explicitly listed in evidence), is a partially hydrogenated pyridine derivative featuring a methyl substituent at the 1-position and a 1,4-dihydro structure. This compound belongs to the dihydropyridine class, which is widely studied for its redox-active properties and applications in asymmetric synthesis, catalysis, and medicinal chemistry. For instance, 3-Pyridineacetic acid, 1,4-dihydro-1-methyl-, methyl ester (CAS: 39998-23-7) shares the 1,4-dihydro-1-methyl core and has a molecular weight of 167 g/mol .
Scientific Research Applications
Medicinal Applications
1,4-Dihydropyridines are known for their diverse therapeutic properties. They serve as essential scaffolds in drug discovery due to their ability to modulate biological activities. Key medicinal applications include:
- Calcium Channel Blockers : 1,4-DHP derivatives are widely recognized as L-type calcium channel blockers, commonly used in treating hypertension and angina pectoris. They help relax blood vessels and reduce heart workload .
- Anticancer Agents : Recent studies indicate that certain 1,4-DHP derivatives exhibit significant antiproliferative effects against various cancer cell lines. For example, compounds synthesized with triazole conjugates demonstrated IC50 values ranging from 0.63±0.05 to 5.68±0.14μM against colorectal adenocarcinoma .
- Antimicrobial Activity : Various 1,4-DHP derivatives have shown promising antibacterial activity against pathogens such as E. coli and S. aureus. For instance, a study reported effective antimicrobial activity at concentrations as low as 4μg/mL .
- Neuroprotective Effects : Some derivatives have been studied for their potential neuroprotective properties, contributing to the development of treatments for neurodegenerative diseases .
Insecticidal Applications
Beyond medicinal uses, 1,4-DHP compounds have been explored for their insecticidal properties. Research indicates that certain derivatives can effectively control pest populations in agricultural settings:
- Agrochemical Applications : A review highlighted the need for more comprehensive studies on the insecticidal applications of 1,4-DHP derivatives. Initial findings suggest they could serve as effective agrochemicals due to their structural diversity and biological activity .
Synthetic Advances
The synthesis of 1,4-DHPs has evolved significantly over the years, leading to the development of novel compounds with enhanced properties:
- Green Chemistry Approaches : Recent synthetic methodologies emphasize environmentally friendly techniques such as one-pot reactions and multi-component reactions (MCR). These methods not only improve yield but also reduce waste and reaction time .
- Diverse Functionalization : Researchers have developed various functionalized 1,4-DHPs through innovative synthetic routes that allow for the introduction of different substituents at key positions on the scaffold .
Table 1: Key Properties of Selected 1,4-DHP Derivatives
Compound Name | Activity Type | IC50 (μM) | Reference |
---|---|---|---|
DHP-Triazole Conjugate | Anticancer | 0.63 | |
DHP-Antibiotic | Antimicrobial | 4 | |
DHP-Calcium Channel Blocker | Cardiovascular | N/A |
Case Study 1: Anticancer Activity
A study conducted by Malhi et al. (2022) synthesized a series of novel 1,4-DHPs with carboxylic moieties that exhibited significant anticancer activity against colorectal cancer cell lines. The findings indicated that these compounds could be developed into effective therapeutic agents due to their low toxicity and high selectivity .
Case Study 2: Insecticidal Efficacy
Research focusing on the insecticidal properties of specific DHP derivatives revealed promising results in controlling agricultural pests. The study emphasized the potential of these compounds as eco-friendly alternatives to traditional pesticides .
Q & A
Basic Research Questions
Q. What are the current synthetic methodologies for 1,4-dihydropyridines, and what challenges persist in achieving mild and direct synthesis?
The synthesis of 1,4-dihydropyridines often involves multicomponent reactions (e.g., Hantzsch reaction) or catalytic cyclization of aldehydes, amines, and β-ketoesters. However, direct methods under mild conditions remain challenging due to sensitivity to oxidation and competing side reactions like aromatization to pyridines . Recent advances include solvent-free mechanochemical approaches using aldehydes, malanonitrile, and diethyl acetylenedicarboxylate, which avoid harsh solvents and reduce reaction times .
Q. How can researchers validate the purity and structural integrity of synthesized 1,4-dihydropyridines?
Key analytical techniques include:
- Mass spectrometry (MS) : To confirm molecular weight and fragmentation patterns (e.g., EPA/NIH Mass Spectral Database for reference spectra) .
- Single-crystal X-ray diffraction : Provides unambiguous structural confirmation and insights into stereoelectronic effects influencing biological activity .
- NMR spectroscopy : Critical for verifying regiochemistry and detecting impurities.
Q. What in vitro assays are suitable for preliminary evaluation of 1,4-dihydropyridine bioactivity?
- Calcium channel modulation : Fluorometric assays using cell lines (e.g., HEK293) transfected with L-type calcium channels .
- Antimicrobial activity : Disk diffusion or microdilution assays against Gram-positive/negative bacteria and fungi .
- Antioxidant capacity : DPPH radical scavenging or FRAP assays to assess redox properties .
Q. How does the thermal stability of 1,4-dihydropyridines impact experimental design?
Thermogravimetric analysis (TGA) reveals decomposition temperatures, which inform storage conditions (e.g., refrigeration under inert gas) and reaction setups (e.g., avoiding high-temperature reflux). Derivatives with electron-withdrawing substituents exhibit enhanced stability against oxidation .
Advanced Research Questions
Q. What mechanistic insights explain contradictory results in 1,4-dihydropyridine-mediated calcium channel modulation?
Divergent outcomes may arise from stereoselective binding to channel subunits or off-target effects on other ion channels (e.g., T-type Ca²⁺ or K⁺ channels). Isotope-labeled analogs and patch-clamp electrophysiology can dissect subtype-specific interactions . Computational docking studies (e.g., using AutoDock Vina) further clarify binding poses .
Q. How can structure-activity relationship (SAR) studies optimize 1,4-dihydropyridine derivatives for selective bioactivity?
- Substituent engineering : Electron-donating groups (e.g., -OCH₃) at the 4-position enhance calcium channel affinity, while bulky aryl groups improve antifungal activity .
- Ring hybridization : Fusion with pyrimidine or pyrrolo[3,4-c]pyridine scaffolds modulates lipophilicity and metabolic stability .
Q. What strategies resolve discrepancies in kinetic data for 1,4-dihydropyridine ligand-exchange reactions?
Time-resolved electrospray ionization mass spectrometry (ESI-MS) and principal component analysis (PCA) quantify exchange rates under varying conditions (e.g., solvent polarity, temperature). For example, pyridine ligand dissociation in chloroform (ΔH = 38.8 kcal/mol) is slower than in acetonitrile due to solvent coordination effects .
Q. How can green chemistry principles be applied to scale 1,4-dihydropyridine synthesis sustainably?
- Solvent-free mechanochemistry : Mortar-and-pestle grinding of aldehydes, amines, and acetylenedicarboxylates achieves 80–95% yields without waste .
- Biocatalytic routes : Lipases or immobilized enzymes catalyze asymmetric reductions of pyridinium salts to dihydropyridines .
Q. What advanced characterization techniques elucidate dynamic behavior of 1,4-dihydropyridines in solution?
- Transient absorption spectroscopy : Probes photodecomposition pathways of prodrug candidates (e.g., Pt(II)-pyridine complexes) .
- Variable-temperature NMR : Detects conformational changes and tautomeric equilibria influenced by substituents .
Q. Methodological Notes
- Contradictory data analysis : Always cross-validate results using orthogonal techniques (e.g., HPLC-MS alongside NMR) and statistically model outliers (e.g., Grubbs’ test) .
- Ethical considerations : Prioritize in silico and in vitro screens before animal testing, adhering to ARRIVE guidelines for translational studies.
Comparison with Similar Compounds
Comparison with Structural Analogs
Structural and Functional Group Variations
Key structural analogs include:
Key Observations :
- Substituent Effects : Electron-donating groups (e.g., methoxy in ) enhance solubility and stabilize aromatic systems, while halogenated derivatives (e.g., bromo/iodo in ) are pivotal in cross-coupling reactions.
- Hydrogenation Impact : The 1,4-dihydro structure in Pyridine, 1,4-dihydro-1-methyl- reduces aromaticity, increasing reactivity compared to fully aromatic pyridines .
Comparison :
- Pyridine, 1,4-dihydro-1-methyl- lacks explicit stereocenter data in the evidence, but its methyl group at C1 likely influences steric and electronic environments during catalysis, analogous to substituent effects observed in .
Commercial Availability and Pricing
- 2,3,6-Trimethoxypyridin-4-ol : $356/g (1 g scale) .
- 5-Chloro-2,3-dimethoxypyridin-4-ol : $500/g (1 g scale) .
Pricing Drivers :
- Halogenation (e.g., chloro, bromo) and methoxy groups increase costs due to synthetic complexity.
- Bulk pricing (25 g) often reduces per-gram costs by 40–60% .
Properties
CAS No. |
33666-44-3 |
---|---|
Molecular Formula |
C6H9N |
Molecular Weight |
95.14 g/mol |
IUPAC Name |
1-methyl-4H-pyridine |
InChI |
InChI=1S/C6H9N/c1-7-5-3-2-4-6-7/h3-6H,2H2,1H3 |
InChI Key |
ZKIJTTNWOULCBY-UHFFFAOYSA-N |
SMILES |
CN1C=CCC=C1 |
Canonical SMILES |
CN1C=CCC=C1 |
Key on ui other cas no. |
33666-44-3 |
Origin of Product |
United States |
Disclaimer and Information on In-Vitro Research Products
Please be aware that all articles and product information presented on BenchChem are intended solely for informational purposes. The products available for purchase on BenchChem are specifically designed for in-vitro studies, which are conducted outside of living organisms. In-vitro studies, derived from the Latin term "in glass," involve experiments performed in controlled laboratory settings using cells or tissues. It is important to note that these products are not categorized as medicines or drugs, and they have not received approval from the FDA for the prevention, treatment, or cure of any medical condition, ailment, or disease. We must emphasize that any form of bodily introduction of these products into humans or animals is strictly prohibited by law. It is essential to adhere to these guidelines to ensure compliance with legal and ethical standards in research and experimentation.