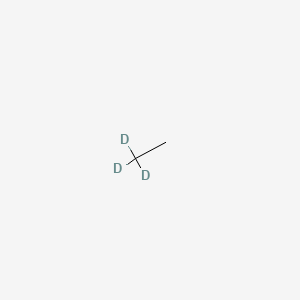
Ethane-1,1,1-d3
Overview
Description
Molecular Structure Analysis
The molecular structure of Ethane-1,1,1-d3 involves two carbon atoms and six hydrogen/deuterium atoms . The infrared spectrum of Ethane-1,1,1-d3 in the 4600–5100-cm−1 and 5600–6300-cm−1 regions has been obtained . Of the eight band centers that were located, only the perpendicular bands ν5 + ν7, at 5065.9 cm−1 and 2ν7, at 5945.5 cm−1, were sufficiently resolved to make a rotational analysis possible .Scientific Research Applications
Cometabolic Biodegradation in Aquifers
Ethane, including its isotopologues like Ethane-1,1,1-d3, has been studied for its potential to stimulate the biological degradation of contaminants like 1,4-dioxane in groundwater aquifers via aerobic cometabolism. Ethane-oxidizing cultures and pure cultures capable of growing on ethane have shown the ability to biodegrade certain environmental contaminants (Hatzinger et al., 2017).
Spectroscopy and Molecular Structure Analysis
Ethane-1,1,1-d3 has been utilized in infra-red and Raman spectroscopy studies. These investigations help in understanding the molecular structure and vibrational spectra of ethane and its isotopologues, contributing to the field of physical chemistry and molecular physics (Stitt, 1939).
Formation of Fragment Ions in Laser Fields
Research involving ethane-1,1,1-d3 includes examining its behavior under intense femtosecond laser fields. This has led to insights into the formation of fragment ions from ethane, contributing to the understanding of molecular dynamics and reaction mechanisms under high-intensity laser irradiation (Schirmel et al., 2013).
Synthesis of Carbon Nanotubes
Ethane has been used as a carbon source in the synthesis of multi-walled carbon nanotubes via chemical vapor deposition. Studies have shown high yield and quality of carbon nanotubes when ethane is used as the feedstock, which is important for materials science and nanotechnology applications (Louis et al., 2005).
Catalytic Cross-Coupling Reactions
Ethane derivatives like 1,1,1-tris(hydroxymethyl)ethane have been explored in copper-catalyzed cross-coupling reactions. These compounds act as efficient ligands in forming C-N, C-S, and C-O bonds, demonstrating their utility in organic synthesis and catalysis (Yao‐Jung Chen & Hsin-Hung Chen, 2006).
Effects in Diesel-Methane Dual-Fuel Combustion
Research on ethane, including its isotopologues, has included the study of its effects on diesel-methane dual-fuel combustion in engines. Adding ethane to methane in dual-fuel combustion systems can enhance ignitability and combustion efficiency, which is significant for automotive and energy research (Ahmad et al., 2021).
Synthesis of Deuterated Alkanes
Ethane-1,1,1-d3 has been synthesized from halides through dehalogenation methods. This process is important in producing deuterated compounds for various scientific applications, including as tracers or in spectroscopic studies (Leblanc et al., 1956).
Photoactive Gold Nanoclusters
The use of ethane-1,1,1-d3 derivatives in the synthesis of gold nanoclusters has been explored. These nanoclusters exhibit interesting properties like photoluminescence and can be used for photogeneration of singlet oxygen, relevant in materials science and photonic applications (Zhang et al., 2018).
properties
IUPAC Name |
1,1,1-trideuterioethane | |
---|---|---|
Source | PubChem | |
URL | https://pubchem.ncbi.nlm.nih.gov | |
Description | Data deposited in or computed by PubChem | |
InChI |
InChI=1S/C2H6/c1-2/h1-2H3/i1D3 | |
Source | PubChem | |
URL | https://pubchem.ncbi.nlm.nih.gov | |
Description | Data deposited in or computed by PubChem | |
InChI Key |
OTMSDBZUPAUEDD-FIBGUPNXSA-N | |
Source | PubChem | |
URL | https://pubchem.ncbi.nlm.nih.gov | |
Description | Data deposited in or computed by PubChem | |
Canonical SMILES |
CC | |
Source | PubChem | |
URL | https://pubchem.ncbi.nlm.nih.gov | |
Description | Data deposited in or computed by PubChem | |
Isomeric SMILES |
[2H]C([2H])([2H])C | |
Source | PubChem | |
URL | https://pubchem.ncbi.nlm.nih.gov | |
Description | Data deposited in or computed by PubChem | |
Molecular Formula |
C2H6 | |
Source | PubChem | |
URL | https://pubchem.ncbi.nlm.nih.gov | |
Description | Data deposited in or computed by PubChem | |
DSSTOX Substance ID |
DTXSID50174200 | |
Record name | Ethane-1,1,1-d3 | |
Source | EPA DSSTox | |
URL | https://comptox.epa.gov/dashboard/DTXSID50174200 | |
Description | DSSTox provides a high quality public chemistry resource for supporting improved predictive toxicology. | |
Molecular Weight |
33.09 g/mol | |
Source | PubChem | |
URL | https://pubchem.ncbi.nlm.nih.gov | |
Description | Data deposited in or computed by PubChem | |
Product Name |
Ethane-1,1,1-d3 | |
CAS RN |
2031-95-0 | |
Record name | Ethane-1,1,1-d3 | |
Source | CAS Common Chemistry | |
URL | https://commonchemistry.cas.org/detail?cas_rn=2031-95-0 | |
Description | CAS Common Chemistry is an open community resource for accessing chemical information. Nearly 500,000 chemical substances from CAS REGISTRY cover areas of community interest, including common and frequently regulated chemicals, and those relevant to high school and undergraduate chemistry classes. This chemical information, curated by our expert scientists, is provided in alignment with our mission as a division of the American Chemical Society. | |
Explanation | The data from CAS Common Chemistry is provided under a CC-BY-NC 4.0 license, unless otherwise stated. | |
Record name | Ethane-1,1,1-d3 | |
Source | ChemIDplus | |
URL | https://pubchem.ncbi.nlm.nih.gov/substance/?source=chemidplus&sourceid=0002031950 | |
Description | ChemIDplus is a free, web search system that provides access to the structure and nomenclature authority files used for the identification of chemical substances cited in National Library of Medicine (NLM) databases, including the TOXNET system. | |
Record name | Ethane-1,1,1-d3 | |
Source | EPA DSSTox | |
URL | https://comptox.epa.gov/dashboard/DTXSID50174200 | |
Description | DSSTox provides a high quality public chemistry resource for supporting improved predictive toxicology. | |
Retrosynthesis Analysis
AI-Powered Synthesis Planning: Our tool employs the Template_relevance Pistachio, Template_relevance Bkms_metabolic, Template_relevance Pistachio_ringbreaker, Template_relevance Reaxys, Template_relevance Reaxys_biocatalysis model, leveraging a vast database of chemical reactions to predict feasible synthetic routes.
One-Step Synthesis Focus: Specifically designed for one-step synthesis, it provides concise and direct routes for your target compounds, streamlining the synthesis process.
Accurate Predictions: Utilizing the extensive PISTACHIO, BKMS_METABOLIC, PISTACHIO_RINGBREAKER, REAXYS, REAXYS_BIOCATALYSIS database, our tool offers high-accuracy predictions, reflecting the latest in chemical research and data.
Strategy Settings
Precursor scoring | Relevance Heuristic |
---|---|
Min. plausibility | 0.01 |
Model | Template_relevance |
Template Set | Pistachio/Bkms_metabolic/Pistachio_ringbreaker/Reaxys/Reaxys_biocatalysis |
Top-N result to add to graph | 6 |
Feasible Synthetic Routes
Q & A
A: The substitution of three hydrogen atoms with deuterium in Ethane-1,1,1-d3 introduces significant changes in its vibrational frequencies compared to regular ethane. This isotopic substitution leads to distinct and identifiable peaks in spectroscopic analyses, allowing researchers to probe molecular structure and dynamics with greater precision. For instance, studies have used Raman spectroscopy [] and infrared spectroscopy [] to analyze the rotational and vibrational characteristics of Ethane-1,1,1-d3, providing insights into its molecular geometry and bonding properties.
A: Researchers have successfully obtained high-resolution infrared spectra of Ethane-1,1,1-d3 []. These spectra have allowed for the identification and analysis of various vibrational bands, including the perpendicular band ν7 [] and the combination bands ν9 + ν10, ν3 + ν4, and ν5 []. Analysis of these bands has yielded important molecular constants such as rotational constants (A0, B0) [] and Coriolis coupling constants (ζ) []. These parameters are crucial for understanding the molecule's rotational-vibrational behavior and overall structure.
A: The incorporation of deuterium, a heavier isotope of hydrogen, results in a kinetic isotope effect. This means that reactions involving the breaking of C-D bonds in Ethane-1,1,1-d3 proceed at a slower rate compared to reactions involving C-H bond cleavage in ethane. This difference in reactivity has been exploited in studies examining the radiolysis of Ethane-1,1,1-d3 []. By analyzing the product distribution at various pressures and temperatures, researchers can gain valuable insights into the reaction mechanisms and energy transfer processes involved.
A: An efficient method for synthesizing Ethane-1,1,1-d3 involves the dehalogenation of 1,1,1-trichloroethane with zinc dust in a deuterium oxide (D2O) and dioxane solution []. This reaction leads to the replacement of chlorine atoms with deuterium, resulting in high yields of Ethane-1,1,1-d3 with good isotopic purity. This synthetic approach highlights the importance of deuterated reagents and solvents in preparing specifically labeled compounds for research purposes.
A: The unique properties of Ethane-1,1,1-d3 make it a valuable tool for studying photochemical reactions. Researchers have investigated the photolysis of Ethane-1,1,1-d3 at specific wavelengths, such as 1470 Å, to understand the photodissociation dynamics and the influence of pressure on the product channels []. These studies shed light on the fundamental processes involved in light-matter interactions and energy transfer within molecules.
Disclaimer and Information on In-Vitro Research Products
Please be aware that all articles and product information presented on BenchChem are intended solely for informational purposes. The products available for purchase on BenchChem are specifically designed for in-vitro studies, which are conducted outside of living organisms. In-vitro studies, derived from the Latin term "in glass," involve experiments performed in controlled laboratory settings using cells or tissues. It is important to note that these products are not categorized as medicines or drugs, and they have not received approval from the FDA for the prevention, treatment, or cure of any medical condition, ailment, or disease. We must emphasize that any form of bodily introduction of these products into humans or animals is strictly prohibited by law. It is essential to adhere to these guidelines to ensure compliance with legal and ethical standards in research and experimentation.