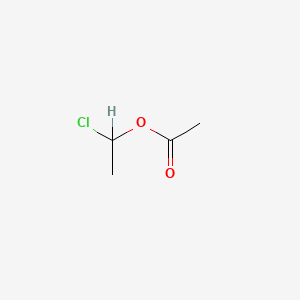
1-Chloroethyl acetate
Overview
Description
1-Chloroethyl acetate is an organic compound with the molecular formula C4H7ClO2. It is a colorless liquid that is used in various chemical processes and applications. The compound is known for its reactivity and is often utilized in organic synthesis.
Preparation Methods
Synthetic Routes and Reaction Conditions: 1-Chloroethyl acetate can be synthesized through the reaction of 1-chloroethanol with acetic anhydride or acetyl chloride. The reaction typically occurs under acidic conditions to facilitate the esterification process.
Industrial Production Methods: In industrial settings, this compound is produced by the esterification of 1-chloroethanol with acetic acid in the presence of a catalyst such as sulfuric acid. The reaction is carried out at elevated temperatures to increase the reaction rate and yield.
Chemical Reactions Analysis
Types of Reactions: 1-Chloroethyl acetate undergoes various chemical reactions, including:
Nucleophilic Substitution: The chlorine atom can be replaced by other nucleophiles such as hydroxide ions, leading to the formation of 1-hydroxyethyl acetate.
Hydrolysis: In the presence of water and an acid or base catalyst, this compound can hydrolyze to produce acetic acid and 1-chloroethanol.
Reduction: The compound can be reduced to form ethyl acetate and hydrogen chloride.
Common Reagents and Conditions:
Nucleophilic Substitution: Reagents such as sodium hydroxide or potassium hydroxide are commonly used under mild conditions.
Hydrolysis: Acidic or basic conditions with water are employed.
Reduction: Reducing agents like lithium aluminum hydride are used under anhydrous conditions.
Major Products Formed:
Nucleophilic Substitution: 1-Hydroxyethyl acetate
Hydrolysis: Acetic acid and 1-chloroethanol
Reduction: Ethyl acetate and hydrogen chloride
Scientific Research Applications
1-Chloroethyl acetate has several applications in scientific research:
Chemistry: It is used as an intermediate in the synthesis of various organic compounds.
Biology: The compound is utilized in the study of enzyme-catalyzed reactions involving ester hydrolysis.
Medicine: It serves as a precursor in the synthesis of pharmaceuticals and other bioactive molecules.
Industry: this compound is employed in the production of solvents, plasticizers, and other industrial chemicals.
Mechanism of Action
The mechanism of action of 1-chloroethyl acetate involves its reactivity as an ester and an alkyl halide. The compound can undergo nucleophilic substitution reactions, where the chlorine atom is replaced by other nucleophiles. This reactivity is due to the electron-withdrawing effect of the chlorine atom, which makes the carbon atom more susceptible to nucleophilic attack.
Comparison with Similar Compounds
1-Chloroethyl acetate can be compared with other similar compounds such as:
Ethyl acetate: Unlike this compound, ethyl acetate does not contain a chlorine atom, making it less reactive in nucleophilic substitution reactions.
1-Bromoethyl acetate: This compound is similar in structure but contains a bromine atom instead of chlorine, which can affect its reactivity and the types of reactions it undergoes.
1-Chloroethyl propionate: This compound has a similar structure but with a different ester group, which can influence its physical and chemical properties.
This compound is unique due to its combination of ester and alkyl halide functionalities, making it a versatile compound in various chemical reactions and applications.
Properties
IUPAC Name |
1-chloroethyl acetate | |
---|---|---|
Source | PubChem | |
URL | https://pubchem.ncbi.nlm.nih.gov | |
Description | Data deposited in or computed by PubChem | |
InChI |
InChI=1S/C4H7ClO2/c1-3(5)7-4(2)6/h3H,1-2H3 | |
Source | PubChem | |
URL | https://pubchem.ncbi.nlm.nih.gov | |
Description | Data deposited in or computed by PubChem | |
InChI Key |
CGKKDGMMKSOGLM-UHFFFAOYSA-N | |
Source | PubChem | |
URL | https://pubchem.ncbi.nlm.nih.gov | |
Description | Data deposited in or computed by PubChem | |
Canonical SMILES |
CC(OC(=O)C)Cl | |
Source | PubChem | |
URL | https://pubchem.ncbi.nlm.nih.gov | |
Description | Data deposited in or computed by PubChem | |
Molecular Formula |
C4H7ClO2 | |
Source | PubChem | |
URL | https://pubchem.ncbi.nlm.nih.gov | |
Description | Data deposited in or computed by PubChem | |
DSSTOX Substance ID |
DTXSID40974559 | |
Record name | 1-Chloroethyl acetate | |
Source | EPA DSSTox | |
URL | https://comptox.epa.gov/dashboard/DTXSID40974559 | |
Description | DSSTox provides a high quality public chemistry resource for supporting improved predictive toxicology. | |
Molecular Weight |
122.55 g/mol | |
Source | PubChem | |
URL | https://pubchem.ncbi.nlm.nih.gov | |
Description | Data deposited in or computed by PubChem | |
CAS No. |
5912-58-3 | |
Record name | Ethanol, 1-chloro-, acetate | |
Source | ChemIDplus | |
URL | https://pubchem.ncbi.nlm.nih.gov/substance/?source=chemidplus&sourceid=0005912583 | |
Description | ChemIDplus is a free, web search system that provides access to the structure and nomenclature authority files used for the identification of chemical substances cited in National Library of Medicine (NLM) databases, including the TOXNET system. | |
Record name | 1-Chloroethyl acetate | |
Source | EPA DSSTox | |
URL | https://comptox.epa.gov/dashboard/DTXSID40974559 | |
Description | DSSTox provides a high quality public chemistry resource for supporting improved predictive toxicology. | |
Record name | 1-chloroethyl acetate | |
Source | European Chemicals Agency (ECHA) | |
URL | https://echa.europa.eu/information-on-chemicals | |
Description | The European Chemicals Agency (ECHA) is an agency of the European Union which is the driving force among regulatory authorities in implementing the EU's groundbreaking chemicals legislation for the benefit of human health and the environment as well as for innovation and competitiveness. | |
Explanation | Use of the information, documents and data from the ECHA website is subject to the terms and conditions of this Legal Notice, and subject to other binding limitations provided for under applicable law, the information, documents and data made available on the ECHA website may be reproduced, distributed and/or used, totally or in part, for non-commercial purposes provided that ECHA is acknowledged as the source: "Source: European Chemicals Agency, http://echa.europa.eu/". Such acknowledgement must be included in each copy of the material. ECHA permits and encourages organisations and individuals to create links to the ECHA website under the following cumulative conditions: Links can only be made to webpages that provide a link to the Legal Notice page. | |
Retrosynthesis Analysis
AI-Powered Synthesis Planning: Our tool employs the Template_relevance Pistachio, Template_relevance Bkms_metabolic, Template_relevance Pistachio_ringbreaker, Template_relevance Reaxys, Template_relevance Reaxys_biocatalysis model, leveraging a vast database of chemical reactions to predict feasible synthetic routes.
One-Step Synthesis Focus: Specifically designed for one-step synthesis, it provides concise and direct routes for your target compounds, streamlining the synthesis process.
Accurate Predictions: Utilizing the extensive PISTACHIO, BKMS_METABOLIC, PISTACHIO_RINGBREAKER, REAXYS, REAXYS_BIOCATALYSIS database, our tool offers high-accuracy predictions, reflecting the latest in chemical research and data.
Strategy Settings
Precursor scoring | Relevance Heuristic |
---|---|
Min. plausibility | 0.01 |
Model | Template_relevance |
Template Set | Pistachio/Bkms_metabolic/Pistachio_ringbreaker/Reaxys/Reaxys_biocatalysis |
Top-N result to add to graph | 6 |
Feasible Synthetic Routes
Disclaimer and Information on In-Vitro Research Products
Please be aware that all articles and product information presented on BenchChem are intended solely for informational purposes. The products available for purchase on BenchChem are specifically designed for in-vitro studies, which are conducted outside of living organisms. In-vitro studies, derived from the Latin term "in glass," involve experiments performed in controlled laboratory settings using cells or tissues. It is important to note that these products are not categorized as medicines or drugs, and they have not received approval from the FDA for the prevention, treatment, or cure of any medical condition, ailment, or disease. We must emphasize that any form of bodily introduction of these products into humans or animals is strictly prohibited by law. It is essential to adhere to these guidelines to ensure compliance with legal and ethical standards in research and experimentation.