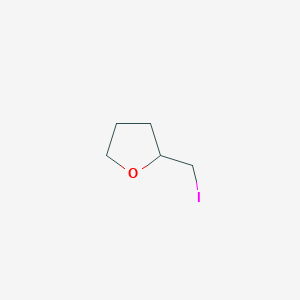
2-(Iodomethyl)tetrahydrofuran
Overview
Description
2-(Iodomethyl)tetrahydrofuran is a chemical compound with the molecular formula C5H9IO . It is used in various chemical reactions and has been the subject of several research studies .
Synthesis Analysis
The synthesis of this compound involves the reaction of 2-allylcyclohexenone derivatives with iodine in a mixture of THF/water . Recent advancements in the stereoselective synthesis of tetrahydrofurans have also been reported, which involve the use of nucleophilic substitution processes .Molecular Structure Analysis
The molecular structure of this compound consists of a five-membered ring with four carbon atoms and one oxygen atom. The compound has an iodomethyl group attached to one of the carbon atoms in the ring .Chemical Reactions Analysis
This compound is involved in various chemical reactions. For instance, it has been used in the formation of 2,3-trans-disubstituted tetrahydrofurans via radical cyclizations of α-thioesters bearing tethered vinylsilane groups .Physical And Chemical Properties Analysis
This compound has a density of 1.8±0.1 g/cm^3, a boiling point of 208.4±13.0 °C at 760 mmHg, and a vapor pressure of 0.3±0.4 mmHg at 25°C . It also has a molar refractivity of 37.7±0.3 cm^3 and a molar volume of 119.7±3.0 cm^3 .Scientific Research Applications
Alternative Solvent in Organic Synthesis
2-(Iodomethyl)tetrahydrofuran and its related compounds have been explored for their potential as alternative solvents in organic chemistry. One variant, 2-Methyltetrahydrofuran (2-MeTHF), derived from renewable resources, has been identified as a promising alternative solvent for environmentally benign synthesis strategies. Its unique properties, such as low miscibility with water, high boiling point, and remarkable stability, make it suitable for use in syntheses involving organometallics, organocatalysis, biotransformations, and processing lignocellulosic materials (Pace et al., 2012).
Synthesis of Halogenated Tetrahydrofurans
Research into the synthesis of bromo- and iodomethyl-substituted tetrahydrofurans has been conducted, involving the use of bis(homoallylic) alcohols. This approach has enabled the creation of halogenated tetrahydrofurans, which are valuable in various synthetic applications. Such methods offer a route to synthesize complex molecules, including those with pharmaceutical relevance (Hartung et al., 2003).
Applications in Asymmetric Synthesis
The compound has been utilized in asymmetric syntheses, particularly in the creation of substituted homoallyl alcohols and chloro-amino sulfones. This indicates its potential in the production of stereochemically complex molecules, which are important in the development of enantioselective drugs and other substances (Rajender & Gais, 2007).
Electrophilic Bifunctional Peroxides in Synthesis
Recent advances include the use of 2-iodomethylallyl peroxides in a [4 + 1] cyclization reaction for synthesizing a broad range of 2,2-disubstituted tetrahydrofurans. This innovative method has opened new avenues for accessing chiral tetrahydrofurans with high diastereoselectivities, which are valuable in pharmaceutical chemistry (Gao et al., 2019).
Catalytic Oxidative Amination
A catalytic oxidative amination of tetrahydrofuran using visible light has been developed. This method activates the C(sp3)-H bond of tetrahydrofuran and allows for the synthesis of N-substituted azoles, providing a green route for the generation of important organic compounds (Zhang et al., 2017).
Safety and Hazards
Future Directions
Future research on 2-(Iodomethyl)tetrahydrofuran could focus on its potential applications in medicinal chemistry and drug design, given its role in the synthesis of biologically active molecules . Additionally, further studies could explore its use in the synthesis of other chemical compounds and its mechanism of action in these reactions .
properties
IUPAC Name |
2-(iodomethyl)oxolane | |
---|---|---|
Source | PubChem | |
URL | https://pubchem.ncbi.nlm.nih.gov | |
Description | Data deposited in or computed by PubChem | |
InChI |
InChI=1S/C5H9IO/c6-4-5-2-1-3-7-5/h5H,1-4H2 | |
Source | PubChem | |
URL | https://pubchem.ncbi.nlm.nih.gov | |
Description | Data deposited in or computed by PubChem | |
InChI Key |
BMZJORGYEOQMFR-UHFFFAOYSA-N | |
Source | PubChem | |
URL | https://pubchem.ncbi.nlm.nih.gov | |
Description | Data deposited in or computed by PubChem | |
Canonical SMILES |
C1CC(OC1)CI | |
Source | PubChem | |
URL | https://pubchem.ncbi.nlm.nih.gov | |
Description | Data deposited in or computed by PubChem | |
Molecular Formula |
C5H9IO | |
Source | PubChem | |
URL | https://pubchem.ncbi.nlm.nih.gov | |
Description | Data deposited in or computed by PubChem | |
DSSTOX Substance ID |
DTXSID40327107 | |
Record name | 2-(iodomethyl)tetrahydrofuran | |
Source | EPA DSSTox | |
URL | https://comptox.epa.gov/dashboard/DTXSID40327107 | |
Description | DSSTox provides a high quality public chemistry resource for supporting improved predictive toxicology. | |
Molecular Weight |
212.03 g/mol | |
Source | PubChem | |
URL | https://pubchem.ncbi.nlm.nih.gov | |
Description | Data deposited in or computed by PubChem | |
CAS RN |
5831-70-9 | |
Record name | 2-(iodomethyl)tetrahydrofuran | |
Source | EPA DSSTox | |
URL | https://comptox.epa.gov/dashboard/DTXSID40327107 | |
Description | DSSTox provides a high quality public chemistry resource for supporting improved predictive toxicology. | |
Record name | 2-(iodomethyl)oxolane | |
Source | European Chemicals Agency (ECHA) | |
URL | https://echa.europa.eu/information-on-chemicals | |
Description | The European Chemicals Agency (ECHA) is an agency of the European Union which is the driving force among regulatory authorities in implementing the EU's groundbreaking chemicals legislation for the benefit of human health and the environment as well as for innovation and competitiveness. | |
Explanation | Use of the information, documents and data from the ECHA website is subject to the terms and conditions of this Legal Notice, and subject to other binding limitations provided for under applicable law, the information, documents and data made available on the ECHA website may be reproduced, distributed and/or used, totally or in part, for non-commercial purposes provided that ECHA is acknowledged as the source: "Source: European Chemicals Agency, http://echa.europa.eu/". Such acknowledgement must be included in each copy of the material. ECHA permits and encourages organisations and individuals to create links to the ECHA website under the following cumulative conditions: Links can only be made to webpages that provide a link to the Legal Notice page. | |
Retrosynthesis Analysis
AI-Powered Synthesis Planning: Our tool employs the Template_relevance Pistachio, Template_relevance Bkms_metabolic, Template_relevance Pistachio_ringbreaker, Template_relevance Reaxys, Template_relevance Reaxys_biocatalysis model, leveraging a vast database of chemical reactions to predict feasible synthetic routes.
One-Step Synthesis Focus: Specifically designed for one-step synthesis, it provides concise and direct routes for your target compounds, streamlining the synthesis process.
Accurate Predictions: Utilizing the extensive PISTACHIO, BKMS_METABOLIC, PISTACHIO_RINGBREAKER, REAXYS, REAXYS_BIOCATALYSIS database, our tool offers high-accuracy predictions, reflecting the latest in chemical research and data.
Strategy Settings
Precursor scoring | Relevance Heuristic |
---|---|
Min. plausibility | 0.01 |
Model | Template_relevance |
Template Set | Pistachio/Bkms_metabolic/Pistachio_ringbreaker/Reaxys/Reaxys_biocatalysis |
Top-N result to add to graph | 6 |
Feasible Synthetic Routes
Disclaimer and Information on In-Vitro Research Products
Please be aware that all articles and product information presented on BenchChem are intended solely for informational purposes. The products available for purchase on BenchChem are specifically designed for in-vitro studies, which are conducted outside of living organisms. In-vitro studies, derived from the Latin term "in glass," involve experiments performed in controlled laboratory settings using cells or tissues. It is important to note that these products are not categorized as medicines or drugs, and they have not received approval from the FDA for the prevention, treatment, or cure of any medical condition, ailment, or disease. We must emphasize that any form of bodily introduction of these products into humans or animals is strictly prohibited by law. It is essential to adhere to these guidelines to ensure compliance with legal and ethical standards in research and experimentation.