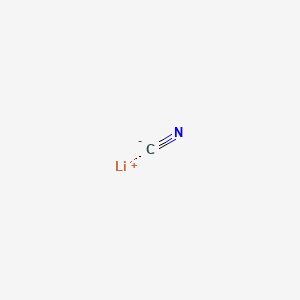
Lithium cyanide
Overview
Description
Lithium cyanide (LiCN) is an inorganic compound composed of lithium (Li⁺) and cyanide (CN⁻) ions. It is a white crystalline solid with a molecular weight of approximately 32.96 g/mol (calculated from atomic masses: Li = 6.94, C = 12.01, N = 14.01). As a metal cyanide, it is highly toxic, requiring stringent safety protocols for handling and disposal .
Preparation Methods
Direct Reaction of Lithium Hydroxide with Hydrogen Cyanide
The most classical and widely reported method for the preparation of lithium cyanide is the direct reaction between lithium hydroxide (LiOH) and hydrogen cyanide (HCN):
$$
\text{LiOH} + \text{HCN} \rightarrow \text{LiCN} + \text{H}_2\text{O}
$$
- This reaction is typically conducted under controlled conditions to safely handle the toxic HCN gas.
- The reaction yields this compound as a white, hygroscopic solid that is soluble in water.
- The process requires careful control of temperature and stoichiometry to avoid side reactions and ensure high purity of LiCN.
Laboratory-Scale Preparation Using Acetone Cyanohydrin
An alternative laboratory-scale synthesis involves acetone cyanohydrin as a surrogate for HCN, reacting with lithium hydride (LiH):
$$
(\text{CH}3)2C(OH)CN + \text{LiH} \rightarrow (\text{CH}3)2CO + \text{LiCN} + \text{H}_2
$$
- This method avoids the direct use of hydrogen cyanide gas, improving safety.
- Acetone cyanohydrin provides the cyanide group, which upon reaction with lithium hydride forms this compound and acetone as a byproduct.
- The hydrogen gas released requires proper ventilation and safety measures.
Catalytic Formation in Organic Synthesis Processes
This compound also appears as an intermediate or catalyst in organic synthesis processes, particularly in the hydrocyanation of unsaturated compounds:
- For example, in the preparation of isophorone nitrile, this compound acts as a catalyst in the reaction of isophorone with hydrogen cyanide.
- The process involves maintaining a low but sufficient concentration of free cyanide to prevent side reactions such as the formation of lithium hydroxide and undesirable byproducts.
- The reaction temperature is controlled between 80°C and 115°C, with continuous feeding of HCN to maintain catalyst activity.
- After the reaction, acidification with a polyacidic acid precipitates lithium salts, which are removed by filtration, followed by vacuum distillation to purify the product and remove excess HCN and isophorone.
This catalytic method highlights the importance of this compound in facilitating cyanation reactions while minimizing impurities and byproducts.
Non-Aqueous Cyanation of Halides Using this compound
This compound is employed in non-aqueous media, such as tetrahydrofuran (THF), to convert organic halides (RX) into nitriles (RCN):
$$
\text{RX} + \text{LiCN} \rightarrow \text{RCN} + \text{LiX}
$$
- This reaction is useful in organic synthesis for introducing the cyanide functional group.
- This compound used in this context is often freshly prepared or commercially available in solution form.
- The reaction conditions avoid aqueous media to prevent hydrolysis and maximize yield.
Summary Table of this compound Preparation Methods
Research Findings and Considerations
- The catalytic use of this compound in hydrocyanation reactions (e.g., isophorone nitrile synthesis) demonstrates the critical balance of cyanide concentration to avoid formation of lithium hydroxide, which leads to byproducts such as diisophorone and its nitrile derivatives.
- The acetone cyanohydrin method is an important laboratory alternative, providing a safer route to LiCN without direct HCN handling, but requires lithium hydride, which is reactive and must be handled carefully.
- Non-aqueous cyanation using this compound in solvents like THF expands the utility of LiCN in organic synthesis, offering high efficiency and selectivity for nitrile formation.
- This compound decomposes upon heating near 600 °C to cyanamide and carbon, and reacts with acids to liberate hydrogen cyanide, necessitating careful storage and handling conditions.
Biological Activity
Lithium cyanide (LiCN) is a compound that has garnered attention due to its potential biological effects and applications. This article explores its biological activity, focusing on toxicity, mechanisms of action, and relevant case studies.
This compound is an inorganic compound consisting of lithium and cyanide ions. It is typically encountered as a white crystalline solid. The chemical formula is represented as LiCN, where lithium serves as a cation and cyanide as an anion. The compound is highly soluble in water, which influences its biological interactions.
Toxicity Profile
This compound exhibits significant toxicity, primarily due to the presence of the cyanide ion, which is known to inhibit cellular respiration by binding to cytochrome c oxidase in the mitochondrial electron transport chain. This inhibition leads to reduced ATP production and can result in cellular hypoxia.
Toxicological Studies
- Acute Toxicity : Studies have shown that exposure to this compound can lead to acute poisoning symptoms, including headache, dizziness, confusion, and in severe cases, respiratory failure or death .
- Chronic Effects : Long-term exposure may result in neurological deficits and other systemic effects due to the compound's interference with normal cellular functions .
The biological activity of this compound can be attributed to several mechanisms:
- Cyanide Ion Toxicity : The cyanide ion acts as a potent inhibitor of cytochrome c oxidase, leading to decreased oxygen utilization in tissues. This mechanism underlies the acute toxicity associated with this compound exposure .
- Oxidative Stress : Lithium compounds are known to induce oxidative stress in cells. Research indicates that this compound can alter the balance between reactive oxygen species (ROS) and antioxidant defenses, contributing to cellular damage and apoptosis .
Case Study 1: Acute Cyanide Poisoning
A documented case involved a patient who ingested this compound. Clinical findings included metabolic acidosis and elevated lactate levels, indicative of anaerobic metabolism due to impaired cellular respiration. Treatment involved administration of hydroxocobalamin, which binds free cyanide ions, facilitating their excretion .
Case Study 2: Environmental Impact
Research has indicated that this compound can contaminate groundwater sources due to improper disposal practices in industrial settings. Studies on aquatic organisms exposed to this compound revealed significant mortality rates and reproductive impairments, highlighting the environmental risks associated with this compound .
Research Findings
Recent investigations into the biological activity of this compound have focused on its genotoxic effects:
- A study examined the impact of lithium compounds on DNA integrity using various assays such as the comet assay and micronucleus test. Results showed that exposure led to significant DNA fragmentation and chromosomal aberrations in cultured cells .
- Another study explored the antioxidant defense mechanisms in cells exposed to this compound, revealing alterations in glutathione levels and increased malondialdehyde (MDA) production, markers of oxidative stress .
Summary Table of Biological Effects
Scientific Research Applications
Chemical Reagent
Lithium cyanide as a Cyanation Agent:
LiCN is primarily used as a reagent for the cyanation of organic compounds. It facilitates the substitution of halogen atoms in organic molecules with cyano groups, making it a valuable tool in synthetic organic chemistry. The general reaction can be represented as follows:
where represents an organic group and is a halogen atom . This reaction is particularly useful for synthesizing nitriles, which are important intermediates in pharmaceuticals and agrochemicals.
Adduct Formation:
this compound can form stable adducts with certain amides, such as dimethylformamide (DMF), enhancing its stability and solubility. These adducts can be utilized to produce LiCN more efficiently from lithium chloride and sodium cyanide under specific conditions, promoting economic production methods .
Lithium Extraction Processes
Mining and Processing:
LiCN plays a crucial role in the extraction of lithium from ores and brines. In particular, it is used in processes that involve the conversion of lithium-bearing minerals into lithium carbonate or lithium hydroxide. The Jadar Valley project in Serbia highlights the potential of lithium mining where LiCN could be involved in processing jadarite ore to extract lithium efficiently .
Advanced Materials
Potential Applications in Battery Technology:
Recent studies have explored the use of LiCN in developing advanced materials for batteries. For instance, research indicates that LiCN could be integrated into cathode materials to enhance performance characteristics. The compound's ability to form covalent bonds with other materials may improve the efficiency of lithium-ion batteries .
Industrial Uses
Metal Processing:
In industrial settings, cyanides, including LiCN, are utilized for enhancing metal ion solubility and facilitating bonding with other ions or molecules. This application is particularly relevant in mining and metal plating industries where LiCN can assist in the extraction of precious metals like gold and silver from ores .
Case Studies and Research Findings
Q & A
Basic Research Questions
Q. What are the key considerations for synthesizing lithium cyanide with high purity in laboratory settings?
- Methodological Answer : Synthesis requires strict control of stoichiometric ratios (Li:CN⁻) in anhydrous conditions due to lithium's hygroscopic nature and cyanide's sensitivity to moisture. Purification involves recrystallization from dry ethanol under inert atmosphere, followed by vacuum drying . Analytical validation (e.g., ion chromatography for cyanide quantification ) ensures purity ≥98%. Safety protocols mandate fume hood use, cyanide-specific training, and emergency decontamination kits .
Q. What safety protocols are essential when handling this compound in experimental setups?
- Methodological Answer : Protocols include:
- Training : Completion of cyanide safety modules covering spill response, first aid (e.g., amyl nitrite administration for acute exposure ), and waste neutralization (using hypochlorite solutions).
- Storage : Segregated, airtight containers labeled with hazard symbols, stored in ventilated cabinets.
- Exposure Controls : Continuous air monitoring for HCN gas and PPE (nitrile gloves, face shields) .
Q. How can researchers accurately quantify cyanide species in this compound solutions using modern analytical techniques?
- Methodological Answer : Automated colorimetric methods (e.g., EPA 335.4) with membrane dialysis minimize interferences from thiocyanates and sulfides. Calibration curves using potassium cyanide standards (0.1–10 ppm range) and quality control via duplicate samples (≤35% RPD) ensure precision . Data validation includes blank subtraction and recovery tests (85–115% acceptable) .
Advanced Research Questions
Q. What methodologies are recommended for determining the thermodynamic stability of this compound under varying environmental conditions?
- Methodological Answer : Use thermogravimetric analysis (TGA) coupled with differential scanning calorimetry (DSC) to measure decomposition temperatures (Td) under controlled humidity (0–80% RH). Compare experimental ΔHf values with computational models (e.g., DFT calculations for lattice energy) to resolve discrepancies in literature-reported stability ranges .
Q. How can contradictory data on the reactivity of this compound with organic solvents be systematically resolved?
- Methodological Answer : Design a matrix of controlled experiments varying solvent polarity (e.g., DMSO vs. THF), temperature (25–60°C), and LiCN concentration. Use <sup>13</sup>C NMR to track cyanide speciation and GC-MS to identify byproducts. Apply multivariate regression to isolate dominant factors (e.g., solvent dielectric constant vs. Lewis acidity) causing discrepancies .
Q. What strategies should be employed to validate this compound experimental data when encountering matrix interferences?
- Methodological Answer : Implement matrix-matched calibration standards and standard addition methods to account for ionic strength effects. For solid-state studies, use synchrotron XRD to differentiate LiCN polymorphs from impurities (e.g., LiOH). Validate with independent techniques like Raman spectroscopy or ICP-OES for cross-correlation .
Q. What are the critical factors in designing a life cycle assessment (LCA) for this compound production processes?
- Methodological Answer : Define system boundaries to include lithium extraction (e.g., brine vs. ore), cyanide synthesis (e.g., Andrussow process), and waste treatment. Ensure consistency in energy mix assumptions (e.g., grid electricity vs. renewables) and allocation methods (mass vs. economic basis) when comparing LCAs. Sensitivity analysis identifies high-impact variables (e.g., HCN leakage rates) .
Q. What computational approaches are most effective for modeling the electronic structure of this compound in solid-state studies?
- Methodological Answer : Plane-wave DFT with van der Waals corrections (e.g., PBE-D3) accurately predicts LiCN’s bandgap and ionic conductivity. Validate with experimental XPS data for valence bands and neutron diffraction for lattice parameters. Open-source codes (e.g., Quantum ESPRESSO) enhance reproducibility .
Q. Data Presentation & Reproducibility
Q. How should large datasets from this compound kinetic studies be presented to ensure clarity and reproducibility?
- Methodological Answer : Raw data (e.g., time-resolved UV-Vis spectra) should be archived in supplementary materials. Processed data in the main text must include error bars (±2σ) and statistical metrics (R<sup>2</sup>, p-values). Follow journal guidelines for figure formatting (e.g., ACS Style) and provide instrument calibration logs .
Q. What steps ensure methodological reproducibility in this compound synthesis and characterization?
Comparison with Similar Compounds
Comparison with Similar Cyanide Compounds
Chemical and Physical Properties
A comparative analysis of lithium cyanide with sodium cyanide (NaCN), potassium cyanide (KCN), and copper(I) cyanide (CuCN) is presented below:
Key Observations :
- Solubility : this compound’s high solubility is comparable to NaCN and KCN but contrasts with CuCN, which is insoluble. The copper-lithium complex exists as a liquid solution, highlighting the role of lithium in stabilizing cyanide complexes .
- Density : this compound has a lower density than NaCN or KCN, reflecting lithium’s smaller ionic radius and lighter atomic mass.
Environmental and Regulatory Considerations
- Regulatory Reporting : Under the U.S. EPA’s Toxics Release Inventory (TRI), this compound is reportable as both a "lithium compound" and "cyanide compound." Threshold determinations require reporting the entire compound weight, whereas releases are quantified based on the cyanide portion .
- Waste Treatment : Biological treatments like Moving Bed Biofilm Reactors (MBBR) effectively degrade cyanide ions in wastewater, though lithium’s persistence may require additional steps for metal recovery .
Research Findings and Industrial Relevance
- Catalytic Limitations : this compound’s inferior performance compared to LiOH in catalysis underscores the impact of counterion selection in synthetic chemistry .
- Thermal Stability : Alkali metal cyanides (LiCN, NaCN, KCN) decompose at high temperatures, releasing HCN gas. This compound’s lower thermal stability (compared to NaCN) limits its use in high-temperature processes .
- Environmental Persistence : Cyanide compounds, including LiCN, are prioritized for remediation due to acute toxicity. The MBBR system achieves near-complete conversion of cyanide to nitrogen gas, offering a sustainable alternative to physical methods like reverse osmosis .
Properties
IUPAC Name |
lithium;cyanide | |
---|---|---|
Source | PubChem | |
URL | https://pubchem.ncbi.nlm.nih.gov | |
Description | Data deposited in or computed by PubChem | |
InChI |
InChI=1S/CN.Li/c1-2;/q-1;+1 | |
Source | PubChem | |
URL | https://pubchem.ncbi.nlm.nih.gov | |
Description | Data deposited in or computed by PubChem | |
InChI Key |
JORQDGTZGKHEEO-UHFFFAOYSA-N | |
Source | PubChem | |
URL | https://pubchem.ncbi.nlm.nih.gov | |
Description | Data deposited in or computed by PubChem | |
Canonical SMILES |
[Li+].[C-]#N | |
Source | PubChem | |
URL | https://pubchem.ncbi.nlm.nih.gov | |
Description | Data deposited in or computed by PubChem | |
Molecular Formula |
LiCN, CLiN | |
Record name | lithium cyanide | |
Source | Wikipedia | |
URL | https://en.wikipedia.org/wiki/Lithium_cyanide | |
Description | Chemical information link to Wikipedia. | |
Source | PubChem | |
URL | https://pubchem.ncbi.nlm.nih.gov | |
Description | Data deposited in or computed by PubChem | |
DSSTOX Substance ID |
DTXSID10946920 | |
Record name | Lithium cyanide | |
Source | EPA DSSTox | |
URL | https://comptox.epa.gov/dashboard/DTXSID10946920 | |
Description | DSSTox provides a high quality public chemistry resource for supporting improved predictive toxicology. | |
Molecular Weight |
33.0 g/mol | |
Source | PubChem | |
URL | https://pubchem.ncbi.nlm.nih.gov | |
Description | Data deposited in or computed by PubChem | |
Physical Description |
Clear brownish-yellow liquid; [MSDSonline] | |
Record name | Lithium cyanide | |
Source | Haz-Map, Information on Hazardous Chemicals and Occupational Diseases | |
URL | https://haz-map.com/Agents/8204 | |
Description | Haz-Map® is an occupational health database designed for health and safety professionals and for consumers seeking information about the adverse effects of workplace exposures to chemical and biological agents. | |
Explanation | Copyright (c) 2022 Haz-Map(R). All rights reserved. Unless otherwise indicated, all materials from Haz-Map are copyrighted by Haz-Map(R). No part of these materials, either text or image may be used for any purpose other than for personal use. Therefore, reproduction, modification, storage in a retrieval system or retransmission, in any form or by any means, electronic, mechanical or otherwise, for reasons other than personal use, is strictly prohibited without prior written permission. | |
CAS No. |
2408-36-8 | |
Record name | Lithium cyanide | |
Source | ChemIDplus | |
URL | https://pubchem.ncbi.nlm.nih.gov/substance/?source=chemidplus&sourceid=0002408368 | |
Description | ChemIDplus is a free, web search system that provides access to the structure and nomenclature authority files used for the identification of chemical substances cited in National Library of Medicine (NLM) databases, including the TOXNET system. | |
Record name | Lithium cyanide | |
Source | EPA DSSTox | |
URL | https://comptox.epa.gov/dashboard/DTXSID10946920 | |
Description | DSSTox provides a high quality public chemistry resource for supporting improved predictive toxicology. | |
Record name | Lithium cyanide | |
Source | European Chemicals Agency (ECHA) | |
URL | https://echa.europa.eu/substance-information/-/substanceinfo/100.017.554 | |
Description | The European Chemicals Agency (ECHA) is an agency of the European Union which is the driving force among regulatory authorities in implementing the EU's groundbreaking chemicals legislation for the benefit of human health and the environment as well as for innovation and competitiveness. | |
Explanation | Use of the information, documents and data from the ECHA website is subject to the terms and conditions of this Legal Notice, and subject to other binding limitations provided for under applicable law, the information, documents and data made available on the ECHA website may be reproduced, distributed and/or used, totally or in part, for non-commercial purposes provided that ECHA is acknowledged as the source: "Source: European Chemicals Agency, http://echa.europa.eu/". Such acknowledgement must be included in each copy of the material. ECHA permits and encourages organisations and individuals to create links to the ECHA website under the following cumulative conditions: Links can only be made to webpages that provide a link to the Legal Notice page. | |
Retrosynthesis Analysis
AI-Powered Synthesis Planning: Our tool employs the Template_relevance Pistachio, Template_relevance Bkms_metabolic, Template_relevance Pistachio_ringbreaker, Template_relevance Reaxys, Template_relevance Reaxys_biocatalysis model, leveraging a vast database of chemical reactions to predict feasible synthetic routes.
One-Step Synthesis Focus: Specifically designed for one-step synthesis, it provides concise and direct routes for your target compounds, streamlining the synthesis process.
Accurate Predictions: Utilizing the extensive PISTACHIO, BKMS_METABOLIC, PISTACHIO_RINGBREAKER, REAXYS, REAXYS_BIOCATALYSIS database, our tool offers high-accuracy predictions, reflecting the latest in chemical research and data.
Strategy Settings
Precursor scoring | Relevance Heuristic |
---|---|
Min. plausibility | 0.01 |
Model | Template_relevance |
Template Set | Pistachio/Bkms_metabolic/Pistachio_ringbreaker/Reaxys/Reaxys_biocatalysis |
Top-N result to add to graph | 6 |
Feasible Synthetic Routes
Disclaimer and Information on In-Vitro Research Products
Please be aware that all articles and product information presented on BenchChem are intended solely for informational purposes. The products available for purchase on BenchChem are specifically designed for in-vitro studies, which are conducted outside of living organisms. In-vitro studies, derived from the Latin term "in glass," involve experiments performed in controlled laboratory settings using cells or tissues. It is important to note that these products are not categorized as medicines or drugs, and they have not received approval from the FDA for the prevention, treatment, or cure of any medical condition, ailment, or disease. We must emphasize that any form of bodily introduction of these products into humans or animals is strictly prohibited by law. It is essential to adhere to these guidelines to ensure compliance with legal and ethical standards in research and experimentation.