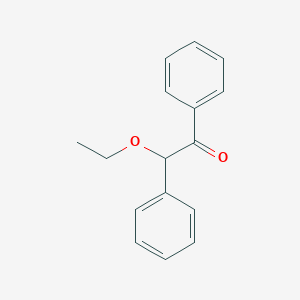
Benzoin ethyl ether
Overview
Description
Benzoin ethyl ether (BEE), chemically known as 2-hydroxy-1,2-diphenylethyl ethyl ether (C₁₆H₁₆O₂), is a derivative of benzoin where the hydroxyl group is replaced by an ethoxy group. It is synthesized via alkylation of benzoin using ethyl iodide and silver oxide under controlled conditions . Recent advancements utilize heterogeneous catalysts like NiAl-layered double hydroxides (NiAl-HTLcs) or Ni(HCO₃)₂, achieving high selectivity (>99%) and conversions up to 54.83% under optimized conditions (75°C, 1 h) . BEE is widely employed as a photoinitiator in polymer chemistry due to its ability to generate free radicals under UV light .
Preparation Methods
Traditional Two-Step Synthesis via Cyanide-Catalyzed Condensation
The conventional route involves two sequential reactions: (1) benzoin formation via benzaldehyde condensation and (2) etherification with ethanol.
Benzoin Condensation
Benzaldehyde undergoes self-condensation in the presence of cyanide ions (NaCN or KCN) to form benzoin. The reaction proceeds via a nucleophilic addition mechanism, where cyanide acts as a catalyst :
6\text{H}5\text{CHO} \xrightarrow{\text{CN}^-} \text{C}6\text{H}5\text{CH(OH)COC}6\text{H}5
Key Parameters :
-
Catalyst : 1–2 wt% NaCN
-
Solvent : Ethanol/water mixture (3:1 ratio)
-
Temperature : 60–70°C
Etherification with Ethanol
Benzoin reacts with excess ethanol under acidic conditions (HCl or H₂SO₄) to form benzoin ethyl ether. Thionyl chloride (SOCl₂) is often used as a dehydrating agent to shift equilibrium toward product formation :
6\text{H}5\text{CH(OH)COC}6\text{H}5 + \text{C}2\text{H}5\text{OH} \xrightarrow{\text{SOCl}2} \text{C}6\text{H}5\text{CH(OEt)COC}6\text{H}5 + \text{H}2\text{O}
Industrial Protocol (Patent CN101712612A) :
Step | Conditions | Ratios (Benzoin : Ethanol : SOCl₂) | Yield |
---|---|---|---|
Etherification | 50–60°C, 4–6 h reflux | 1 : 1.4 : 0.5 | 82% |
Crude Product Workup | Water washing, phase separation | 15:1 (water : crude mixture) | – |
Recrystallization | Ethanol + activated carbon, 60–80°C | 1 : 1.5 (crude : ethanol) | 95% |
Advantages :
Limitations :
Acid-Catalyzed One-Pot Synthesis
Recent patents describe a one-pot method using thionyl chloride as both catalyst and dehydrating agent, eliminating the need for cyanide .
Reaction Mechanism
Thionyl chloride activates the hydroxyl group of benzoin, facilitating nucleophilic attack by ethanol:
2 \rightarrow \text{Chlorobenzoin intermediate} \xrightarrow{\text{EtOH}} \text{this compound} + \text{HCl} + \text{SO}2
Optimization Data
Comparative results from patent embodiments :
Embodiment | Temperature (°C) | Reaction Time (h) | SOCl₂ (mol%) | Yield |
---|---|---|---|---|
1 | 50 | 4 | 50 | 82% |
2 | 55 | 5 | 50 | 85% |
3 | 60 | 6 | 50 | 88% |
Critical Factors :
-
Temperature : Higher temperatures (60°C) improve kinetics but risk side reactions.
-
Molar Ratios : Excess ethanol (1.4:1 ethanol:benzoin) ensures complete conversion .
Alternative Catalytic Systems
Hydrotalcite-Based Catalysts
Hydrotalcite (Mg₆Al₂(OH)₁₆CO₃·4H₂O) has been explored as a non-toxic alternative to cyanide. However, yields remain suboptimal :
Challenges :
-
Low Brønsted acidity reduces etherification efficiency.
-
Catalyst regeneration complicates industrial adoption.
Bismuth Oxychloride Catalysts
A 2019 study reported BiOCl as a metal thermal catalyst for one-step synthesis from benzaldehyde and ethanol :
Catalyst Loading | Temperature (°C) | Time (h) | Yield |
---|---|---|---|
5 wt% BiOCl | 120 | 12 | 68% |
Advantages :
Industrial Considerations
Environmental Impact
Method | E-Factor* | Key Waste Streams |
---|---|---|
Cyanide + SOCl₂ | 8.2 | CN⁻, HCl, SO₂ |
One-Pot (SOCl₂) | 5.7 | HCl, SO₂ |
BiOCl Catalyzed | 3.1 | BiOCl sludge |
Scientific Research Applications
Applications in Scientific Research
Benzoin ethyl ether has a broad spectrum of applications across several scientific fields:
Chemistry
- Photoinitiator in Polymerization:
Application | Description |
---|---|
UV-Curable Coatings | Used to initiate polymerization reactions that create durable coatings. |
Inks | Facilitates the curing process in printing applications. |
Adhesives | Enhances bonding strength through polymerization. |
Biology
- Role in Photochemical Reactions:
Industrial Applications
- Manufacturing Processes:
Case Studies
Case Study 1: Photoinitiation Mechanism
A study conducted on the efficiency of this compound as a photoinitiator revealed that it effectively initiates polymerization at specific wavelengths of UV light. The research demonstrated that varying the concentration of this compound can significantly influence the rate of polymer formation and the mechanical properties of the resulting materials.
Case Study 2: Biological Activity Exploration
Research investigating the biological activities of this compound highlighted its potential applications in drug delivery systems. The compound's ability to facilitate drug release upon exposure to UV light was emphasized, suggesting its utility in targeted therapies .
Mechanism of Action
The primary mechanism of action for benzoin ethyl ether involves its role as a photosensitizer. Upon exposure to ultraviolet light, it undergoes photochemical decomposition to generate free radicals. These radicals can initiate polymerization reactions, making it an effective photoinitiator in various applications .
Comparison with Similar Compounds
Structural and Physical Properties
Benzoin derivatives share a common 1,2-diphenylethane backbone but differ in substituents. Key physical properties are summarized below:
Compound | Molecular Formula | Melting Point (°C) | Key Functional Group |
---|---|---|---|
Benzoin | C₁₄H₁₂O₂ | 132–135 | Hydroxyl, ketone |
Benzoin methyl ether | C₁₅H₁₄O₂ | 47.5–48.5 | Methoxy, ketone |
Benzoin ethyl ether | C₁₆H₁₆O₂ | 56–59 | Ethoxy, ketone |
Benzoin isopropyl ether | C₁₇H₁₈O₂ | 76–77 | Isopropoxy, ketone |
BEE exhibits a higher melting point than its methyl analog (BME) due to increased van der Waals interactions from the ethyl group .
Stability and Byproduct Formation
BEE is more stable than hydrobenzoin derivatives but less stable than benzoin under acidic conditions. Its synthesis often generates ethyl benzoate as a byproduct via oxidation, a challenge mitigated by catalytic methods .
Data Tables
Table 2. Photolysis Products of Benzoin Ethers in Silicalite
Compound | Major Products | Yield (%) |
---|---|---|
Benzoin methyl ether | Pinacol methyl ether | 21.5 |
This compound | Benzaldehyde, benzoic acid | 18.7 |
Benzoin isopropyl ether | Rearranged ketones | 15.2 |
Biological Activity
Benzoin ethyl ether, also known as α-ethoxy-α-phenylacetophenone, is an organic compound with the formula CHO. It is primarily recognized for its applications in organic synthesis and photochemistry. This article explores its biological activity, including its synthesis, potential therapeutic uses, and mechanisms of action.
- Molecular Weight : 240.30 g/mol
- Melting Point : 59-61 °C
- Structure : The compound features an ethoxy group attached to a benzoin structure, which contributes to its reactivity and biological properties.
Synthesis
This compound can be synthesized through various methods, including:
- One-Pot Synthesis : Recent studies have demonstrated a highly selective one-pot synthesis using NiAlCe-hydrotalcite catalysts, achieving over 98% selectivity with significant conversion rates of benzaldehyde .
- Catalytic Reactions : Heterogeneous catalysts such as Ni(HCO₃)₂ have shown high catalytic activity in the formation of this compound from benzaldehyde and ethanol .
Antimicrobial Properties
This compound has been evaluated for its antimicrobial activity. In vitro studies indicate that it exhibits significant antibacterial effects against various strains of bacteria. For instance, research has shown that it can inhibit the growth of Staphylococcus aureus and Escherichia coli, suggesting potential applications in developing antimicrobial agents .
Cytotoxic Effects
The cytotoxicity of this compound has been investigated in several cell lines. A study revealed that it induces apoptosis in cancer cell lines through the activation of caspase pathways, indicating its potential as an anticancer agent. The compound demonstrated a dose-dependent response, with higher concentrations leading to increased cell death .
Photochemical Activity
This compound is known for its photochemical properties. Under UV light exposure, it can undergo photolysis to produce reactive intermediates that may have biological implications. This property is particularly relevant in the context of photodynamic therapy (PDT), where light-activated compounds are used to treat cancer .
Case Studies
- Antimicrobial Efficacy : A study assessed the effectiveness of this compound against bacterial biofilms. The results indicated a reduction in biofilm formation by 70% at certain concentrations, highlighting its potential use in medical devices to prevent infections .
- Cytotoxic Mechanism : Research involving human cancer cell lines demonstrated that this compound triggers apoptosis via mitochondrial pathways. The study utilized flow cytometry to analyze cell cycle arrest and apoptosis markers, confirming its role as a potential chemotherapeutic agent .
Research Findings Summary Table
Q & A
Basic Research Questions
Q. How can researchers optimize the synthesis of BEE to achieve high purity and yield?
- Methodology : Use a two-step condensation reaction involving benzaldehyde and ethyl ether derivatives under alkaline conditions. Monitor reaction progress via thin-layer chromatography (TLC) and purify via recrystallization from ethanol or methanol to remove unreacted precursors . Adjust molar ratios (e.g., benzaldehyde:ethyl ether = 1:1.2) and reaction time (12–24 hours at 60–80°C) to minimize side products like desyl chloride.
Q. What safety protocols are critical when handling BEE in laboratory settings?
- Methodology : Classify BEE as a Category 9 environmental hazard (UN 3077) due to its ecotoxicity. Use fume hoods to avoid inhalation of vapors (melting point: 59–61°C; boiling point: 194–195°C at 20 mmHg). Employ personal protective equipment (PPE), including nitrile gloves and lab coats, and store in airtight containers away from oxidizing agents .
Q. Which analytical techniques are most effective for validating BEE’s structural integrity and purity?
- Methodology :
- Nuclear Magnetic Resonance (NMR) : Confirm the ethoxy (–OCH₂CH₃) and acetophenone (C=O) groups via characteristic peaks (¹H NMR: δ 1.3–1.5 ppm for –CH₃; 13C NMR: δ 207 ppm for ketone) .
- High-Performance Liquid Chromatography (HPLC) : Use a C18 column with UV detection (λ = 254 nm) to assess purity >99% .
- Melting Point Analysis : Verify consistency with literature values (59–61°C) to detect impurities .
Advanced Research Questions
Q. How do substituent groups in benzoin derivatives influence chiral recognition in chromatographic separations?
- Methodology : Compare BEE (ethoxy group) with analogs like benzoin methyl ether (BME) and desyl chloride. Under normal-phase (NP) HPLC, steric hindrance from the ethoxy group reduces enantioselectivity (α = 1.0 for BEE vs. α = 1.6 for BME). Use polysaccharide-based chiral stationary phases (CSPs) and polar organic mode (POP) eluents to enhance resolution .
Q. What reaction mechanisms explain byproduct formation during BEE-mediated photopolymerization?
- Methodology : Investigate radical initiation pathways using UV light (λ = 365 nm). Byproducts like benzaldehyde arise from α-cleavage of the excited ketone group. Characterize intermediates via electron paramagnetic resonance (EPR) spectroscopy and optimize monomer-to-initiator ratios to suppress side reactions .
Q. How can contradictions in chiral separation efficiency under different eluent conditions be resolved?
- Methodology : In micellar electrokinetic chromatography (MEKC), BEE’s resolution varies with ionic liquid (IL) modifiers. For example, 1-butyl-3-methylimidazolium tetrafluoroborate (BMIMBF₄) increases hydrophobic interactions, improving separation. Contrast results with aqueous buffer systems and validate using poly-sodium oleyl-L-leucyl-valinate (poly-L-SOLV) as a pseudo-stationary phase .
Q. Which computational models predict BEE’s reactivity in radical-mediated reactions?
- Methodology : Apply density functional theory (DFT) to calculate bond dissociation energies (BDEs) for the C–O and C=O bonds. Correlate with experimental data from photolysis studies to identify dominant cleavage pathways. Software tools like Gaussian 16 with B3LYP/6-31G* basis sets are recommended .
Q. How does BEE interact with ionic liquid modifiers in MEKC to enhance separation selectivity?
- Methodology : Evaluate ILs (e.g., BMIMBF₄) as mobile phase additives. Measure changes in electroosmotic flow (EOF) and partitioning behavior using capillary electrophoresis (CE). ILs improve solubility of hydrophobic analytes and modulate surfactant aggregation, enhancing resolution of BEE enantiomers .
Q. Tables for Key Data
Property | BEE Value | Reference |
---|---|---|
Molecular Formula | C₁₆H₁₆O₂ | |
Melting Point | 59–61°C | |
Chiral Resolution (α, NP-HPLC) | 1.0 (vs. 1.6 for BME) | |
Environmental Hazard Class | UN 3077 (Category 9) |
Properties
IUPAC Name |
2-ethoxy-1,2-diphenylethanone | |
---|---|---|
Source | PubChem | |
URL | https://pubchem.ncbi.nlm.nih.gov | |
Description | Data deposited in or computed by PubChem | |
InChI |
InChI=1S/C16H16O2/c1-2-18-16(14-11-7-4-8-12-14)15(17)13-9-5-3-6-10-13/h3-12,16H,2H2,1H3 | |
Source | PubChem | |
URL | https://pubchem.ncbi.nlm.nih.gov | |
Description | Data deposited in or computed by PubChem | |
InChI Key |
KMNCBSZOIQAUFX-UHFFFAOYSA-N | |
Source | PubChem | |
URL | https://pubchem.ncbi.nlm.nih.gov | |
Description | Data deposited in or computed by PubChem | |
Canonical SMILES |
CCOC(C1=CC=CC=C1)C(=O)C2=CC=CC=C2 | |
Source | PubChem | |
URL | https://pubchem.ncbi.nlm.nih.gov | |
Description | Data deposited in or computed by PubChem | |
Molecular Formula |
C16H16O2 | |
Source | PubChem | |
URL | https://pubchem.ncbi.nlm.nih.gov | |
Description | Data deposited in or computed by PubChem | |
DSSTOX Substance ID |
DTXSID40862220 | |
Record name | Ethanone, 2-ethoxy-1,2-diphenyl- | |
Source | EPA DSSTox | |
URL | https://comptox.epa.gov/dashboard/DTXSID40862220 | |
Description | DSSTox provides a high quality public chemistry resource for supporting improved predictive toxicology. | |
Molecular Weight |
240.30 g/mol | |
Source | PubChem | |
URL | https://pubchem.ncbi.nlm.nih.gov | |
Description | Data deposited in or computed by PubChem | |
Physical Description |
White crystalline powder; [Alfa Aesar MSDS] | |
Record name | Benzoin ethyl ether | |
Source | Haz-Map, Information on Hazardous Chemicals and Occupational Diseases | |
URL | https://haz-map.com/Agents/20296 | |
Description | Haz-Map® is an occupational health database designed for health and safety professionals and for consumers seeking information about the adverse effects of workplace exposures to chemical and biological agents. | |
Explanation | Copyright (c) 2022 Haz-Map(R). All rights reserved. Unless otherwise indicated, all materials from Haz-Map are copyrighted by Haz-Map(R). No part of these materials, either text or image may be used for any purpose other than for personal use. Therefore, reproduction, modification, storage in a retrieval system or retransmission, in any form or by any means, electronic, mechanical or otherwise, for reasons other than personal use, is strictly prohibited without prior written permission. | |
CAS No. |
574-09-4 | |
Record name | (±)-Benzoin ethyl ether | |
Source | CAS Common Chemistry | |
URL | https://commonchemistry.cas.org/detail?cas_rn=574-09-4 | |
Description | CAS Common Chemistry is an open community resource for accessing chemical information. Nearly 500,000 chemical substances from CAS REGISTRY cover areas of community interest, including common and frequently regulated chemicals, and those relevant to high school and undergraduate chemistry classes. This chemical information, curated by our expert scientists, is provided in alignment with our mission as a division of the American Chemical Society. | |
Explanation | The data from CAS Common Chemistry is provided under a CC-BY-NC 4.0 license, unless otherwise stated. | |
Record name | Ethanone, 2-ethoxy-1,2-diphenyl- | |
Source | ChemIDplus | |
URL | https://pubchem.ncbi.nlm.nih.gov/substance/?source=chemidplus&sourceid=0000574094 | |
Description | ChemIDplus is a free, web search system that provides access to the structure and nomenclature authority files used for the identification of chemical substances cited in National Library of Medicine (NLM) databases, including the TOXNET system. | |
Record name | Ethanone, 2-ethoxy-1,2-diphenyl- | |
Source | EPA Chemicals under the TSCA | |
URL | https://www.epa.gov/chemicals-under-tsca | |
Description | EPA Chemicals under the Toxic Substances Control Act (TSCA) collection contains information on chemicals and their regulations under TSCA, including non-confidential content from the TSCA Chemical Substance Inventory and Chemical Data Reporting. | |
Record name | Ethanone, 2-ethoxy-1,2-diphenyl- | |
Source | EPA DSSTox | |
URL | https://comptox.epa.gov/dashboard/DTXSID40862220 | |
Description | DSSTox provides a high quality public chemistry resource for supporting improved predictive toxicology. | |
Record name | 2-ethoxy-1,2-diphenylethan-1-one | |
Source | European Chemicals Agency (ECHA) | |
URL | https://echa.europa.eu/substance-information/-/substanceinfo/100.008.516 | |
Description | The European Chemicals Agency (ECHA) is an agency of the European Union which is the driving force among regulatory authorities in implementing the EU's groundbreaking chemicals legislation for the benefit of human health and the environment as well as for innovation and competitiveness. | |
Explanation | Use of the information, documents and data from the ECHA website is subject to the terms and conditions of this Legal Notice, and subject to other binding limitations provided for under applicable law, the information, documents and data made available on the ECHA website may be reproduced, distributed and/or used, totally or in part, for non-commercial purposes provided that ECHA is acknowledged as the source: "Source: European Chemicals Agency, http://echa.europa.eu/". Such acknowledgement must be included in each copy of the material. ECHA permits and encourages organisations and individuals to create links to the ECHA website under the following cumulative conditions: Links can only be made to webpages that provide a link to the Legal Notice page. | |
Synthesis routes and methods
Procedure details
Retrosynthesis Analysis
AI-Powered Synthesis Planning: Our tool employs the Template_relevance Pistachio, Template_relevance Bkms_metabolic, Template_relevance Pistachio_ringbreaker, Template_relevance Reaxys, Template_relevance Reaxys_biocatalysis model, leveraging a vast database of chemical reactions to predict feasible synthetic routes.
One-Step Synthesis Focus: Specifically designed for one-step synthesis, it provides concise and direct routes for your target compounds, streamlining the synthesis process.
Accurate Predictions: Utilizing the extensive PISTACHIO, BKMS_METABOLIC, PISTACHIO_RINGBREAKER, REAXYS, REAXYS_BIOCATALYSIS database, our tool offers high-accuracy predictions, reflecting the latest in chemical research and data.
Strategy Settings
Precursor scoring | Relevance Heuristic |
---|---|
Min. plausibility | 0.01 |
Model | Template_relevance |
Template Set | Pistachio/Bkms_metabolic/Pistachio_ringbreaker/Reaxys/Reaxys_biocatalysis |
Top-N result to add to graph | 6 |
Feasible Synthetic Routes
Disclaimer and Information on In-Vitro Research Products
Please be aware that all articles and product information presented on BenchChem are intended solely for informational purposes. The products available for purchase on BenchChem are specifically designed for in-vitro studies, which are conducted outside of living organisms. In-vitro studies, derived from the Latin term "in glass," involve experiments performed in controlled laboratory settings using cells or tissues. It is important to note that these products are not categorized as medicines or drugs, and they have not received approval from the FDA for the prevention, treatment, or cure of any medical condition, ailment, or disease. We must emphasize that any form of bodily introduction of these products into humans or animals is strictly prohibited by law. It is essential to adhere to these guidelines to ensure compliance with legal and ethical standards in research and experimentation.