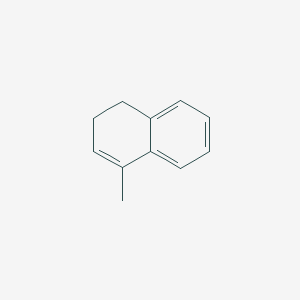
4-Methyl-1,2-dihydronaphthalene
Overview
Description
4-Methyl-1,2-dihydronaphthalene is a chemical compound with the molecular formula C11H12 . Its molecular weight is 144.2130 . The IUPAC name for this compound is Naphthalene, 1,2-dihydro-4-methyl- .
Synthesis Analysis
The synthesis of dihydronaphthalenes, including 4-Methyl-1,2-dihydronaphthalene, has been a subject of research. Literature methods for the preparation of dihydronaphthalenes and benzofluorenes have been described . Efforts to discover new synthetic methods for the synthesis of dihydronaphthalenes and benzofluorenes are ongoing . One method involves the direct single-electron oxidation of methylenecyclopropanes (MCPs) for the rapid construction of 4-aryl-1,2-dihydronaphthalene derivatives by merging visible light photoredox catalysis and cobalt catalysis .
Molecular Structure Analysis
The molecular structure of 4-Methyl-1,2-dihydronaphthalene can be viewed using Java or Javascript . It is also available as a 2D Mol file or as a computed 3D SD file .
Chemical Reactions Analysis
Dihydronaphthalenes, including 4-Methyl-1,2-dihydronaphthalene, are known as useful building blocks in organic synthesis . They can undergo bromination, cyclopropanation, dipolar cycloaddition, and epoxidation reactions to afford useful products .
Physical And Chemical Properties Analysis
4-Methyl-1,2-dihydronaphthalene has a density of 1.0±0.1 g/cm3 . Its boiling point is 220.2±15.0 °C at 760 mmHg . The vapour pressure is 0.2±0.2 mmHg at 25°C . The enthalpy of vaporization is 43.8±0.8 kJ/mol . The flash point is 80.3±9.0 °C . The index of refraction is 1.555 .
Scientific Research Applications
Synthesis of Biologically Active Cyclic Molecules
4-Methyl-1,2-dihydronaphthalene derivatives serve as crucial starting materials for synthesizing biologically active cyclic molecules. Traditional synthetic approaches to these compounds have been extensively reported, highlighting their significance in medicinal chemistry .
Fluorescent Ligands for Estrogen Receptors
These derivatives are utilized as fluorescent ligands for estrogen receptors. Their ability to bind selectively and with high affinity makes them valuable tools for studying receptor binding and assisting in the development of therapeutic agents .
Hepatitis C NS5B Polymerase Inhibitors
The compound exhibits activity as inhibitors of the Hepatitis C NS5B polymerase. This application is particularly important in the search for new treatments against Hepatitis C, a virus affecting millions worldwide .
Inhibitors of Aldosterone Synthase
Recent studies have found 4-Methyl-1,2-dihydronaphthalene derivatives to be potent and selective inhibitors of aldosterone synthase (CYP11B2). This is crucial for the treatment of conditions like congestive heart failure and myocardial fibrosis .
Organic Synthesis Building Blocks
In organic synthesis, these derivatives are known as versatile building blocks. They can undergo various reactions such as bromination, cyclopropanation, dipolar cycloaddition, and epoxidation to afford a range of useful products .
Development of Therapeutic Agents
The presence of the 1,2-dihydronaphthalene ring system in natural products of therapeutic importance, such as cannabisins and negundin B, underscores its value in the development of new therapeutic agents .
Chemical Intermediate in Synthesis
4-Methyl-1,2-dihydronaphthalene serves as a chemical intermediate in the synthesis of more complex chemical entities. Its reactivity and stability under various conditions make it a compound of choice for multi-step synthesis processes .
Future Directions
Future directions in the study of 4-Methyl-1,2-dihydronaphthalene could involve further exploration of its synthesis methods, particularly those involving electrochemical oxidative conditions . Additionally, the transformation of polysubstituted 1,2-dihydronaphthalenes to polysubstituted 1,2,3,4-tetrahydronaphthalenes and polysubstituted naphthalenes shows great potential in synthetic applications .
Mechanism of Action
Target of Action
Dihydronaphthalene derivatives, a class to which this compound belongs, have been found to be potent and selective inhibitors of aldosterone synthase (cyp11b2), a key enzyme involved in the biosynthesis of the hormone aldosterone . They are also used as fluorescent ligands for the estrogen receptor .
Mode of Action
Dihydronaphthalene derivatives are known to interact with their targets, such as the estrogen receptor, by binding to the receptor’s active site, thereby modulating its activity .
Biochemical Pathways
Given that dihydronaphthalene derivatives can inhibit aldosterone synthase (cyp11b2), it can be inferred that they may impact the biosynthesis of aldosterone, a hormone that regulates sodium and potassium balance in the body .
Pharmacokinetics
The molecular weight of the compound is 1442130 , which is within the optimal range for oral bioavailability in drug design
Result of Action
Dihydronaphthalene derivatives have been found to exhibit activity as hepatitis c ns5b polymerase inhibitors
properties
IUPAC Name |
4-methyl-1,2-dihydronaphthalene | |
---|---|---|
Source | PubChem | |
URL | https://pubchem.ncbi.nlm.nih.gov | |
Description | Data deposited in or computed by PubChem | |
InChI |
InChI=1S/C11H12/c1-9-5-4-7-10-6-2-3-8-11(9)10/h2-3,5-6,8H,4,7H2,1H3 | |
Source | PubChem | |
URL | https://pubchem.ncbi.nlm.nih.gov | |
Description | Data deposited in or computed by PubChem | |
InChI Key |
NACZXFJTTCJDJR-UHFFFAOYSA-N | |
Source | PubChem | |
URL | https://pubchem.ncbi.nlm.nih.gov | |
Description | Data deposited in or computed by PubChem | |
Canonical SMILES |
CC1=CCCC2=CC=CC=C12 | |
Source | PubChem | |
URL | https://pubchem.ncbi.nlm.nih.gov | |
Description | Data deposited in or computed by PubChem | |
Molecular Formula |
C11H12 | |
Source | PubChem | |
URL | https://pubchem.ncbi.nlm.nih.gov | |
Description | Data deposited in or computed by PubChem | |
DSSTOX Substance ID |
DTXSID70342484 | |
Record name | 4-Methyl-1,2-dihydronaphthalene | |
Source | EPA DSSTox | |
URL | https://comptox.epa.gov/dashboard/DTXSID70342484 | |
Description | DSSTox provides a high quality public chemistry resource for supporting improved predictive toxicology. | |
Molecular Weight |
144.21 g/mol | |
Source | PubChem | |
URL | https://pubchem.ncbi.nlm.nih.gov | |
Description | Data deposited in or computed by PubChem | |
Product Name |
4-Methyl-1,2-dihydronaphthalene | |
CAS RN |
4373-13-1 | |
Record name | 1,2-Dihydro-4-methylnaphthalene | |
Source | ChemIDplus | |
URL | https://pubchem.ncbi.nlm.nih.gov/substance/?source=chemidplus&sourceid=0004373131 | |
Description | ChemIDplus is a free, web search system that provides access to the structure and nomenclature authority files used for the identification of chemical substances cited in National Library of Medicine (NLM) databases, including the TOXNET system. | |
Record name | 4-Methyl-1,2-dihydronaphthalene | |
Source | EPA DSSTox | |
URL | https://comptox.epa.gov/dashboard/DTXSID70342484 | |
Description | DSSTox provides a high quality public chemistry resource for supporting improved predictive toxicology. | |
Record name | 1,2-DIHYDRO-4-METHYLNAPHTHALENE | |
Source | FDA Global Substance Registration System (GSRS) | |
URL | https://gsrs.ncats.nih.gov/ginas/app/beta/substances/MKS17FP7YF | |
Description | The FDA Global Substance Registration System (GSRS) enables the efficient and accurate exchange of information on what substances are in regulated products. Instead of relying on names, which vary across regulatory domains, countries, and regions, the GSRS knowledge base makes it possible for substances to be defined by standardized, scientific descriptions. | |
Explanation | Unless otherwise noted, the contents of the FDA website (www.fda.gov), both text and graphics, are not copyrighted. They are in the public domain and may be republished, reprinted and otherwise used freely by anyone without the need to obtain permission from FDA. Credit to the U.S. Food and Drug Administration as the source is appreciated but not required. | |
Synthesis routes and methods I
Procedure details
Synthesis routes and methods II
Procedure details
Retrosynthesis Analysis
AI-Powered Synthesis Planning: Our tool employs the Template_relevance Pistachio, Template_relevance Bkms_metabolic, Template_relevance Pistachio_ringbreaker, Template_relevance Reaxys, Template_relevance Reaxys_biocatalysis model, leveraging a vast database of chemical reactions to predict feasible synthetic routes.
One-Step Synthesis Focus: Specifically designed for one-step synthesis, it provides concise and direct routes for your target compounds, streamlining the synthesis process.
Accurate Predictions: Utilizing the extensive PISTACHIO, BKMS_METABOLIC, PISTACHIO_RINGBREAKER, REAXYS, REAXYS_BIOCATALYSIS database, our tool offers high-accuracy predictions, reflecting the latest in chemical research and data.
Strategy Settings
Precursor scoring | Relevance Heuristic |
---|---|
Min. plausibility | 0.01 |
Model | Template_relevance |
Template Set | Pistachio/Bkms_metabolic/Pistachio_ringbreaker/Reaxys/Reaxys_biocatalysis |
Top-N result to add to graph | 6 |
Feasible Synthetic Routes
Q & A
Q1: What are the primary photochemical reactions of 4-MDHN under UV irradiation in solution?
A1: When 4-MDHN is irradiated with 254 nm light in hexane solution, it undergoes several photochemical reactions. The major products identified include:
- 2-(o-tolyl)-1,3-butadiene (T13B) []
- 1-methylbenzobicyclo[3.1.0]hex-2-ene (1-MBBH) []
- 1-methyl-1,4-dihydronaphthalene (1-M-1,4-DHN) []
- 1-methyltetralin (1-MT) []
- 1-methylnaphthalene (1-MN) []
Q2: How does the photochemistry of 4-MDHN differ in the gas phase compared to solution?
A2: The gas-phase photochemistry of 4-MDHN, while similar to solution-phase reactions, shows a dependence on vibrational energy levels. The formation of benzocyclobutene derivatives, not observed in solution, is proposed to occur through either a 2+2 cycloaddition of a vibrationally relaxed o-quinodimethane intermediate or a [, ] sigmatropic shift from specific vibrational levels of the excited state (S1 vib) [].
Q3: What is the role of triplet sensitization in the photochemistry of 4-MDHN?
A3: Triplet sensitization experiments, where energy is transferred from a triplet sensitizer to 4-MDHN, primarily yield small amounts of 1-methylnaphthalene (1-MN). This observation suggests that the direct photolysis reactions, leading to the diverse product distribution, primarily occur through singlet excited state chemistry [].
Q4: What analytical techniques were employed to study the photochemical reactions of 4-MDHN?
A4: While the specific analytical techniques are not detailed within the provided abstracts, product identification and quantification in photochemical studies are commonly achieved using techniques like gas chromatography-mass spectrometry (GC-MS), nuclear magnetic resonance (NMR) spectroscopy, and UV-Vis spectroscopy. Quantum yield measurements, reported for various products, suggest the use of quantitative analytical methods for determining product formation efficiency [, ].
Disclaimer and Information on In-Vitro Research Products
Please be aware that all articles and product information presented on BenchChem are intended solely for informational purposes. The products available for purchase on BenchChem are specifically designed for in-vitro studies, which are conducted outside of living organisms. In-vitro studies, derived from the Latin term "in glass," involve experiments performed in controlled laboratory settings using cells or tissues. It is important to note that these products are not categorized as medicines or drugs, and they have not received approval from the FDA for the prevention, treatment, or cure of any medical condition, ailment, or disease. We must emphasize that any form of bodily introduction of these products into humans or animals is strictly prohibited by law. It is essential to adhere to these guidelines to ensure compliance with legal and ethical standards in research and experimentation.