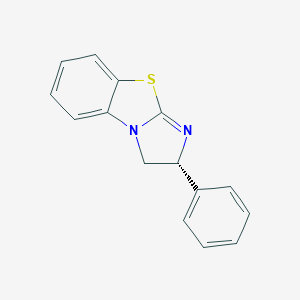
(+)-Benzotetramisole
Overview
Description
+-Benzotetramisole is an organic compound with the chemical formula C19H20N2O2. It is a colorless, crystalline solid that is soluble in organic solvents. It is an important intermediate in the synthesis of various pharmaceuticals, such as anti-inflammatory drugs, antibiotics, and anti-cancer agents. It is also used as a reagent in organic synthesis. (+)-Benzotetramisole is a versatile and important compound that has a wide range of applications in the pharmaceutical, agricultural, and industrial fields.
Scientific Research Applications
Catalytic Asymmetric Rearrangements and Synthesis
(+)-Benzotetramisole has been identified as a catalyst promoting asymmetric rearrangements and syntheses in various chemical processes. For instance, it has been used in the catalytic asymmetric [2,3]-rearrangement of allylic quaternary ammonium salts, yielding syn-α-amino acid derivatives with exceptional diastereo- and enantioselectivity (West et al., 2014). Additionally, benzotetramisole has served as an enantioselective acyl transfer catalyst in the kinetic resolution of secondary benzylic alcohols, demonstrating its versatile catalytic abilities (Birman & Li, 2006). Furthermore, its catalytic role extends to the kinetic resolution of propargylic alcohols, achieving high selectivity factors (Birman & Guo, 2006) and to the kinetic resolution of N-acyl-β-lactams for the production of β-amino acid derivatives (Bumbu & Birman, 2011).
Understanding Mechanisms of Enantioselectivity
Research has also delved into understanding the underlying mechanisms of enantioselectivity in chemical reactions facilitated by benzotetramisole. Density Functional Theory (DFT) calculations have been employed to investigate the origins of enantioselectivity in benzotetramisole-catalyzed dynamic kinetic resolution of azlactones. These studies shed light on the molecular interactions and conformations that lead to high enantioselectivity (Liu et al., 2012).
Recyclable and Immobilized Catalysts
The development of sustainable and efficient catalytic systems is crucial in modern chemistry. Benzotetramisole's application extends to the creation of a polystyrene-supported, enantiopure analogue, allowing for its use as a highly active and enantioselective catalyst in domino Michael addition/cyclization reactions. This analogue facilitates the production of dihydropyridinones with excellent yields and enantioselectivity, demonstrating the potential for recyclable and immobilized catalytic systems (Izquierdo & Pericàs, 2016).
Synthetic Transformations
Benzotetramisole's role in synthetic chemistry is underscored by its involvement in the kinetic resolution of various substrates, leading to the efficient synthesis of chiral compounds. An example includes the benzotetramisole-catalyzed kinetic resolution of 2H-azirines, which provides an efficient method for synthesizing chiral isomers with broad scope, good yield, and high enantioselectivity. The resulting chiral 2H-azirine products are valuable building blocks for further synthetic transformations (Peng et al., 2020).
properties
IUPAC Name |
(2R)-2-phenyl-1,2-dihydroimidazo[2,1-b][1,3]benzothiazole | |
---|---|---|
Source | PubChem | |
URL | https://pubchem.ncbi.nlm.nih.gov | |
Description | Data deposited in or computed by PubChem | |
InChI |
InChI=1S/C15H12N2S/c1-2-6-11(7-3-1)12-10-17-13-8-4-5-9-14(13)18-15(17)16-12/h1-9,12H,10H2/t12-/m0/s1 | |
Source | PubChem | |
URL | https://pubchem.ncbi.nlm.nih.gov | |
Description | Data deposited in or computed by PubChem | |
InChI Key |
YGCWPCVAVSIFLO-LBPRGKRZSA-N | |
Source | PubChem | |
URL | https://pubchem.ncbi.nlm.nih.gov | |
Description | Data deposited in or computed by PubChem | |
Canonical SMILES |
C1C(N=C2N1C3=CC=CC=C3S2)C4=CC=CC=C4 | |
Source | PubChem | |
URL | https://pubchem.ncbi.nlm.nih.gov | |
Description | Data deposited in or computed by PubChem | |
Isomeric SMILES |
C1[C@H](N=C2N1C3=CC=CC=C3S2)C4=CC=CC=C4 | |
Source | PubChem | |
URL | https://pubchem.ncbi.nlm.nih.gov | |
Description | Data deposited in or computed by PubChem | |
Molecular Formula |
C15H12N2S | |
Source | PubChem | |
URL | https://pubchem.ncbi.nlm.nih.gov | |
Description | Data deposited in or computed by PubChem | |
DSSTOX Substance ID |
DTXSID60468566 | |
Record name | (+)-Benzotetramisole | |
Source | EPA DSSTox | |
URL | https://comptox.epa.gov/dashboard/DTXSID60468566 | |
Description | DSSTox provides a high quality public chemistry resource for supporting improved predictive toxicology. | |
Molecular Weight |
252.3 g/mol | |
Source | PubChem | |
URL | https://pubchem.ncbi.nlm.nih.gov | |
Description | Data deposited in or computed by PubChem | |
CAS RN |
885051-07-0 | |
Record name | (+)-Benzotetramisole | |
Source | EPA DSSTox | |
URL | https://comptox.epa.gov/dashboard/DTXSID60468566 | |
Description | DSSTox provides a high quality public chemistry resource for supporting improved predictive toxicology. | |
Retrosynthesis Analysis
AI-Powered Synthesis Planning: Our tool employs the Template_relevance Pistachio, Template_relevance Bkms_metabolic, Template_relevance Pistachio_ringbreaker, Template_relevance Reaxys, Template_relevance Reaxys_biocatalysis model, leveraging a vast database of chemical reactions to predict feasible synthetic routes.
One-Step Synthesis Focus: Specifically designed for one-step synthesis, it provides concise and direct routes for your target compounds, streamlining the synthesis process.
Accurate Predictions: Utilizing the extensive PISTACHIO, BKMS_METABOLIC, PISTACHIO_RINGBREAKER, REAXYS, REAXYS_BIOCATALYSIS database, our tool offers high-accuracy predictions, reflecting the latest in chemical research and data.
Strategy Settings
Precursor scoring | Relevance Heuristic |
---|---|
Min. plausibility | 0.01 |
Model | Template_relevance |
Template Set | Pistachio/Bkms_metabolic/Pistachio_ringbreaker/Reaxys/Reaxys_biocatalysis |
Top-N result to add to graph | 6 |
Feasible Synthetic Routes
Q & A
Q1: What is (+)-Benzotetramisole (BTM) primarily known for in the realm of organic chemistry?
A1: this compound (BTM) has earned recognition as an efficient and versatile chiral acyl-transfer catalyst. [] It has been successfully employed in various enantioselective transformations, including kinetic resolution of alcohols and amines, desymmetrization reactions, and asymmetric cycloadditions. [, , , , , , ]
Q2: How does this compound (BTM) facilitate enantioselective esterification reactions?
A2: this compound (BTM) catalyzes enantioselective esterification through a mechanism involving the activation of carboxylic acids. It forms a chiral acylammonium ion intermediate with the acid anhydride, which then undergoes nucleophilic attack by the alcohol, resulting in the formation of a chiral ester. The chiral environment provided by this compound (BTM) during this process leads to preferential formation of one enantiomer over the other. [, , ]
Q3: What makes this compound (BTM) a versatile catalyst for kinetic resolution? Can you provide specific examples?
A3: this compound (BTM) excels in kinetic resolution due to its ability to selectively acylate one enantiomer of a racemic mixture, leaving the other untouched. This is particularly useful for preparing enantioenriched alcohols, amines, and other valuable chiral building blocks. For instance, this compound (BTM) has been successfully employed in the kinetic resolution of secondary benzylic alcohols, [, , ] propargylic alcohols, [] 2H-azirines, [] cyclic hydroxamic acids, [] and N-acyl-β-lactams. []
Q4: How effective is this compound (BTM) in catalyzing the kinetic resolution of challenging substrates like propargylic alcohols?
A4: this compound (BTM) exhibits remarkable efficiency in the kinetic resolution of propargylic alcohols, achieving selectivity factors of up to 32. This is noteworthy as it represents one of the highest selectivities achieved for this class of substrates using non-enzymatic catalysts. []
Q5: Besides kinetic resolution, what other asymmetric transformations can this compound (BTM) catalyze?
A5: this compound (BTM) goes beyond kinetic resolution, demonstrating its versatility in other asymmetric transformations. For example, it has been employed in the desymmetrization of meso-diol lobelanidine, highlighting its potential in synthesizing complex natural products. [] Moreover, this compound (BTM) has proven effective in dynamic kinetic resolution of azlactones, providing access to di(1-naphthyl)methyl esters of α-amino acids with high enantiomeric excess. []
Q6: How does this compound (BTM) contribute to the synthesis of α-amino acid derivatives?
A6: this compound (BTM) plays a crucial role in the synthesis of α-amino acid derivatives via a tandem palladium and isothiourea relay catalysis. It catalyzes the enantioselective [, ]-sigmatropic rearrangement of allylic ammonium salts, generated in situ from N,N-disubstituted glycine aryl esters and allylic phosphates, to afford syn-α-amino acid derivatives with high diastereo- and enantioselectivity. []
Q7: What are the advantages of using this compound (BTM) in tandem catalytic processes?
A7: Tandem catalytic processes employing this compound (BTM) offer several advantages, including increased efficiency and selectivity. For example, in the tandem palladium and this compound (BTM) catalyzed synthesis of α-amino acid derivatives, the use of this compound (BTM) eliminates the need to isolate the intermediate allylic ammonium salt, streamlining the process and enhancing overall efficiency. []
Q8: Has this compound (BTM) been explored for its potential in flow chemistry applications?
A8: Yes, a polystyrene-supported analogue of this compound (BTM) has been developed and successfully applied in a continuous flow setup for the enantioselective domino Michael addition/cyclization reaction. This immobilized version of this compound (BTM) allows for easy catalyst separation and recycling, making it suitable for large-scale synthesis and industrial applications. []
Q9: What is the molecular formula and molecular weight of this compound (BTM)?
A9: The molecular formula of this compound (BTM) is C15H12N2S, and its molecular weight is 252.33 g/mol. []
Q10: How do structural modifications on the this compound (BTM) scaffold affect its catalytic activity and selectivity?
A10: Studies have shown that structural modifications on the this compound (BTM) scaffold can significantly impact its catalytic activity and selectivity. For instance, introducing substituents on the aromatic ring or modifying the chiral center can influence the steric and electronic properties of the catalyst, thereby affecting its ability to differentiate between enantiomers during reactions. [] Additionally, replacing the benzothiazole moiety with other heterocycles or modifying the N-substituent can also lead to changes in catalytic activity and selectivity. [, ]
Q11: Have computational methods been employed to elucidate the mechanism of this compound (BTM) catalyzed reactions?
A11: Yes, Density Functional Theory (DFT) calculations have been instrumental in understanding the mechanism of this compound (BTM)-catalyzed reactions. For example, DFT studies have provided insights into the transition states involved in the kinetic resolution of azlactones, revealing the importance of electrostatic interactions in enantioselectivity. [] These calculations help optimize reaction conditions and design more efficient catalysts.
Q12: Are there any other catalysts that can be used as alternatives to this compound (BTM) for similar reactions?
A12: While this compound (BTM) is a highly effective catalyst for various asymmetric transformations, other catalysts, such as chiral DMAP derivatives, proline derivatives, and other isothiourea catalysts, have also shown promising results in similar reactions. [, ] The choice of catalyst often depends on the specific substrate and reaction conditions.
Disclaimer and Information on In-Vitro Research Products
Please be aware that all articles and product information presented on BenchChem are intended solely for informational purposes. The products available for purchase on BenchChem are specifically designed for in-vitro studies, which are conducted outside of living organisms. In-vitro studies, derived from the Latin term "in glass," involve experiments performed in controlled laboratory settings using cells or tissues. It is important to note that these products are not categorized as medicines or drugs, and they have not received approval from the FDA for the prevention, treatment, or cure of any medical condition, ailment, or disease. We must emphasize that any form of bodily introduction of these products into humans or animals is strictly prohibited by law. It is essential to adhere to these guidelines to ensure compliance with legal and ethical standards in research and experimentation.