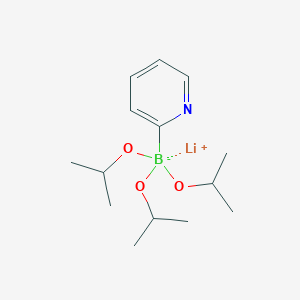
Lithium triisopropyl 2-pyridylborate
Overview
Description
Lithium triisopropyl 2-pyridylborate is an organoboron compound with the molecular formula C14H25BLiNO3. It is a valuable reagent in organic synthesis, particularly in Suzuki-Miyaura cross-coupling reactions. This compound is known for its stability and effectiveness in forming carbon-carbon bonds, making it a crucial component in the synthesis of various organic compounds, including those with potential pharmaceutical applications .
Mechanism of Action
Target of Action
Lithium Triisopropyl 2-Pyridylborate (LT2PB) is a complex compound with a unique mechanism of action. Lithium, a component of lt2pb, is known to interact with several targets, including inositol monophosphatase (impa) and glycogen synthase kinase-3 (gsk-3) .
Mode of Action
Lithium, a component of lt2pb, is known to act as a competitive inhibitor of numerous mg+±dependent factors, transporters, and enzymes . It is proposed that lithium’s therapeutic effects occur indirectly by augmenting a cascade of protective “fail-safe” pathways pre-configured to activate in response to a dangerous low cell [Mg++] situation .
Biochemical Pathways
Lithium is known to inhibit impa and gsk-3, which are involved in the phosphoinositide and wnt/β-catenin signaling pathways, respectively .
Result of Action
Lithium is known to have pervasive protective actions, providing indirect evidence to support the existence of a set of latent, low cell [mg++]-sensitive cell protection systems .
Biochemical Analysis
Biochemical Properties
Lithium triisopropyl 2-pyridylborate plays a significant role in biochemical reactions, particularly in the Suzuki-Miyaura coupling reactions. This compound interacts with various enzymes, proteins, and other biomolecules, facilitating the formation of carbon-carbon bonds. The nature of these interactions involves the coordination of the borate group with the active sites of enzymes, enhancing the catalytic efficiency of the reactions. Additionally, this compound can act as a ligand, forming complexes with metal ions that are crucial for enzymatic activities .
Cellular Effects
This compound influences various types of cells and cellular processes. It has been observed to affect cell signaling pathways, gene expression, and cellular metabolism. The compound can modulate the activity of key signaling molecules, leading to alterations in cellular responses. For instance, it can enhance or inhibit the phosphorylation of proteins involved in signal transduction pathways, thereby affecting downstream cellular events. Furthermore, this compound can influence gene expression by interacting with transcription factors and other regulatory proteins .
Molecular Mechanism
The molecular mechanism of action of this compound involves its binding interactions with biomolecules. The compound can inhibit or activate enzymes by binding to their active sites or allosteric sites. This binding can lead to conformational changes in the enzymes, affecting their catalytic activities. Additionally, this compound can modulate gene expression by interacting with DNA or RNA, influencing the transcription and translation processes. These molecular interactions are crucial for the compound’s biochemical and cellular effects .
Temporal Effects in Laboratory Settings
In laboratory settings, the effects of this compound can change over time. The compound’s stability and degradation are important factors that influence its long-term effects on cellular function. Studies have shown that this compound remains stable under specific conditions, but prolonged exposure to certain environments can lead to its degradation. This degradation can result in the loss of its biochemical activity and potential adverse effects on cells. Long-term studies in vitro and in vivo have provided insights into the temporal dynamics of this compound’s effects .
Dosage Effects in Animal Models
The effects of this compound vary with different dosages in animal models. At low doses, the compound can exhibit beneficial effects, such as enhancing enzymatic activities and modulating cellular processes. At high doses, it can lead to toxic or adverse effects, including cellular damage and disruption of metabolic pathways. Threshold effects have been observed, where the compound’s impact on cellular function changes significantly beyond a certain dosage. These findings highlight the importance of optimizing the dosage of this compound for its safe and effective use .
Metabolic Pathways
This compound is involved in various metabolic pathways, interacting with enzymes and cofactors that regulate metabolic flux and metabolite levels. The compound can influence carbohydrate and nucleotide metabolism by modulating the activity of key enzymes in these pathways. For example, it can inhibit glycogen synthase kinase 3-beta (GSK-3β), leading to increased glycogen synthesis and altered glucose metabolism. Additionally, this compound can affect nucleotide metabolism by interacting with enzymes involved in DNA and RNA synthesis .
Transport and Distribution
The transport and distribution of this compound within cells and tissues are mediated by specific transporters and binding proteins. The compound can be actively transported across cell membranes, facilitated by transporter proteins that recognize its structure. Once inside the cells, this compound can bind to intracellular proteins, influencing its localization and accumulation. These interactions are crucial for the compound’s cellular effects and its ability to reach target sites within the cells .
Subcellular Localization
This compound exhibits specific subcellular localization, which can affect its activity and function. The compound can be directed to specific compartments or organelles within the cells through targeting signals or post-translational modifications. For instance, it can localize to the nucleus, where it interacts with DNA and transcription factors, influencing gene expression. Additionally, this compound can be targeted to the mitochondria, affecting cellular metabolism and energy production .
Preparation Methods
Synthetic Routes and Reaction Conditions
Lithium triisopropyl 2-pyridylborate can be synthesized through a one-pot lithiation, borylation, and subsequent Suzuki-Miyaura coupling of various heterocycles with aryl halides. The process involves the following steps :
Lithiation: The starting material, typically an aryl halide, undergoes lithium-halogen exchange to form a reactive nucleophilic arene.
Borylation: The nucleophilic arene is then quenched in situ with triisopropylborate to form the lithium salt.
Suzuki-Miyaura Coupling: The lithium triisopropyl borate is then used in a Suzuki-Miyaura coupling reaction with an aryl or heteroaryl halide in the presence of a palladium catalyst.
Industrial Production Methods
Industrial production of this compound follows similar synthetic routes but on a larger scale. The process is optimized for higher yields and purity, often involving automated systems for precise control of reaction conditions and efficient handling of reagents .
Chemical Reactions Analysis
Types of Reactions
Lithium triisopropyl 2-pyridylborate primarily undergoes substitution reactions, particularly in Suzuki-Miyaura cross-coupling reactions. These reactions involve the formation of carbon-carbon bonds between the 2-pyridyl group of the reagent and an aryl or heteroaryl halide .
Common Reagents and Conditions
Solvent: Common solvents include tetrahydrofuran (THF) and dimethylformamide (DMF).
Temperature: Reactions are typically conducted at room temperature or slightly elevated temperatures to ensure optimal reaction rates and yields.
Major Products
Scientific Research Applications
Lithium triisopropyl 2-pyridylborate has a wide range of applications in scientific research, including :
Chemistry: Used as a reagent in Suzuki-Miyaura cross-coupling reactions to form carbon-carbon bonds.
Biology: Employed in the synthesis of biologically active molecules and potential drug candidates.
Medicine: Utilized in the development of pharmaceutical compounds with therapeutic potential.
Industry: Applied in the production of advanced materials and specialty chemicals.
Comparison with Similar Compounds
Similar Compounds
Lithium 2-pyridyltriolborate: This compound is structurally similar to lithium triisopropyl 2-pyridylborate, with a triolborate group replacing the triisopropylborate moiety.
2-Bromopyridine: A common starting material for the synthesis of this compound.
4,4″-Disubstituted-2,2′6′,2″-terpyridines: These compounds contain a terpyridine core and are synthesized using similar Suzuki-Miyaura cross-coupling reactions.
Uniqueness
This compound is unique due to its stability and effectiveness in forming carbon-carbon bonds in Suzuki-Miyaura cross-coupling reactions. Its ability to act as a nucleophile in these reactions, coupled with its compatibility with various functional groups, makes it a valuable reagent in organic synthesis .
Properties
IUPAC Name |
lithium;tri(propan-2-yloxy)-pyridin-2-ylboranuide | |
---|---|---|
Source | PubChem | |
URL | https://pubchem.ncbi.nlm.nih.gov | |
Description | Data deposited in or computed by PubChem | |
InChI |
InChI=1S/C14H25BNO3.Li/c1-11(2)17-15(18-12(3)4,19-13(5)6)14-9-7-8-10-16-14;/h7-13H,1-6H3;/q-1;+1 | |
Source | PubChem | |
URL | https://pubchem.ncbi.nlm.nih.gov | |
Description | Data deposited in or computed by PubChem | |
InChI Key |
TWTLFOWAEPOZKO-UHFFFAOYSA-N | |
Source | PubChem | |
URL | https://pubchem.ncbi.nlm.nih.gov | |
Description | Data deposited in or computed by PubChem | |
Canonical SMILES |
[Li+].[B-](C1=CC=CC=N1)(OC(C)C)(OC(C)C)OC(C)C | |
Source | PubChem | |
URL | https://pubchem.ncbi.nlm.nih.gov | |
Description | Data deposited in or computed by PubChem | |
Molecular Formula |
C14H25BLiNO3 | |
Source | PubChem | |
URL | https://pubchem.ncbi.nlm.nih.gov | |
Description | Data deposited in or computed by PubChem | |
DSSTOX Substance ID |
DTXSID50648012 | |
Record name | Lithium tris(propan-2-olato)(pyridin-2-yl)borate(1-) | |
Source | EPA DSSTox | |
URL | https://comptox.epa.gov/dashboard/DTXSID50648012 | |
Description | DSSTox provides a high quality public chemistry resource for supporting improved predictive toxicology. | |
Molecular Weight |
273.1 g/mol | |
Source | PubChem | |
URL | https://pubchem.ncbi.nlm.nih.gov | |
Description | Data deposited in or computed by PubChem | |
CAS No. |
213764-22-8 | |
Record name | Lithium tris(propan-2-olato)(pyridin-2-yl)borate(1-) | |
Source | EPA DSSTox | |
URL | https://comptox.epa.gov/dashboard/DTXSID50648012 | |
Description | DSSTox provides a high quality public chemistry resource for supporting improved predictive toxicology. | |
Record name | 213764-22-8 | |
Source | European Chemicals Agency (ECHA) | |
URL | https://echa.europa.eu/information-on-chemicals | |
Description | The European Chemicals Agency (ECHA) is an agency of the European Union which is the driving force among regulatory authorities in implementing the EU's groundbreaking chemicals legislation for the benefit of human health and the environment as well as for innovation and competitiveness. | |
Explanation | Use of the information, documents and data from the ECHA website is subject to the terms and conditions of this Legal Notice, and subject to other binding limitations provided for under applicable law, the information, documents and data made available on the ECHA website may be reproduced, distributed and/or used, totally or in part, for non-commercial purposes provided that ECHA is acknowledged as the source: "Source: European Chemicals Agency, http://echa.europa.eu/". Such acknowledgement must be included in each copy of the material. ECHA permits and encourages organisations and individuals to create links to the ECHA website under the following cumulative conditions: Links can only be made to webpages that provide a link to the Legal Notice page. | |
Retrosynthesis Analysis
AI-Powered Synthesis Planning: Our tool employs the Template_relevance Pistachio, Template_relevance Bkms_metabolic, Template_relevance Pistachio_ringbreaker, Template_relevance Reaxys, Template_relevance Reaxys_biocatalysis model, leveraging a vast database of chemical reactions to predict feasible synthetic routes.
One-Step Synthesis Focus: Specifically designed for one-step synthesis, it provides concise and direct routes for your target compounds, streamlining the synthesis process.
Accurate Predictions: Utilizing the extensive PISTACHIO, BKMS_METABOLIC, PISTACHIO_RINGBREAKER, REAXYS, REAXYS_BIOCATALYSIS database, our tool offers high-accuracy predictions, reflecting the latest in chemical research and data.
Strategy Settings
Precursor scoring | Relevance Heuristic |
---|---|
Min. plausibility | 0.01 |
Model | Template_relevance |
Template Set | Pistachio/Bkms_metabolic/Pistachio_ringbreaker/Reaxys/Reaxys_biocatalysis |
Top-N result to add to graph | 6 |
Feasible Synthetic Routes
Disclaimer and Information on In-Vitro Research Products
Please be aware that all articles and product information presented on BenchChem are intended solely for informational purposes. The products available for purchase on BenchChem are specifically designed for in-vitro studies, which are conducted outside of living organisms. In-vitro studies, derived from the Latin term "in glass," involve experiments performed in controlled laboratory settings using cells or tissues. It is important to note that these products are not categorized as medicines or drugs, and they have not received approval from the FDA for the prevention, treatment, or cure of any medical condition, ailment, or disease. We must emphasize that any form of bodily introduction of these products into humans or animals is strictly prohibited by law. It is essential to adhere to these guidelines to ensure compliance with legal and ethical standards in research and experimentation.