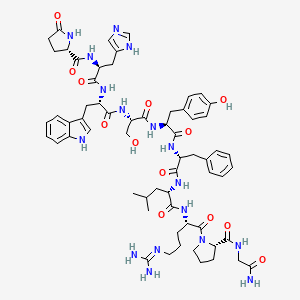
LHRH, Phe(6)-
Overview
Description
Luteinizing hormone-releasing hormone, phenylalanine(6)- (LHRH, Phe(6)-) is a synthetic analogue of the naturally occurring gonadotropin-releasing hormone (GnRH). Both LHRH and GnRH are linear decapeptides, but the key difference lies in the amino acid at position 6: glycine in GnRH and phenylalanine in LHRH, Phe(6)- . This substitution confers agonistic activity to the compound, making it a potent stimulator of the release of luteinizing hormone (LH) and follicle-stimulating hormone (FSH) from the anterior pituitary gland .
Preparation Methods
Synthetic Routes and Reaction Conditions: The synthesis of LHRH, Phe(6)- involves solid-phase peptide synthesis (SPPS), a method commonly used for the production of peptides. The process begins with the attachment of the C-terminal amino acid to a solid resin. Sequential addition of protected amino acids follows, with each cycle involving deprotection and coupling steps. The phenylalanine residue is introduced at the sixth position during this sequence .
Industrial Production Methods: Industrial production of LHRH, Phe(6)- typically employs automated peptide synthesizers to ensure high yield and purity. The synthesized peptide is then cleaved from the resin and purified using high-performance liquid chromatography (HPLC). The final product undergoes rigorous quality control to confirm its identity and purity .
Chemical Reactions Analysis
Types of Reactions: LHRH, Phe(6)- primarily undergoes enzymatic degradation rather than traditional chemical reactions like oxidation or reduction. The primary degradation pathway involves the cleavage of peptide bonds by proteolytic enzymes .
Common Reagents and Conditions: The enzymatic degradation of LHRH, Phe(6)- occurs in the plasma and various tissues. Proteases such as chymotrypsin and elastase are responsible for breaking down the peptide into smaller oligopeptides .
Major Products Formed: The degradation products of LHRH, Phe(6)- are oligopeptides with a maximum of five amino acids. These fragments do not exhibit any GnRH activity and are further degraded or excreted via the kidneys .
Scientific Research Applications
LHRH, Phe(6)- has a wide range of applications in scientific research, particularly in the fields of endocrinology and reproductive biology. It is used to study the regulation of LH and FSH release and to investigate the mechanisms of action of GnRH analogues . In veterinary medicine, LHRH, Phe(6)- is employed to correct reproductive disorders in animals and for zootechnical purposes .
Mechanism of Action
LHRH, Phe(6)- exerts its effects by binding to GnRH receptors on the surface of pituitary gonadotrophs. This binding triggers a cascade of intracellular events, leading to the release of LH and FSH . The activation of these receptors involves the coupling of G proteins and the subsequent activation of adenylate cyclase, resulting in increased cyclic AMP levels . This increase in cyclic AMP ultimately leads to the secretion of LH and FSH .
Comparison with Similar Compounds
Similar Compounds:
- Luteinizing hormone-releasing hormone (GnRH)
- Luteinizing hormone-releasing hormone, D-tryptophan(6)- (LHRH, Trp(6)-)
- Luteinizing hormone-releasing hormone, D-serine(6)- (LHRH, Ser(6)-)
Uniqueness: The primary distinction between LHRH, Phe(6)- and other GnRH analogues lies in the substitution at the sixth position. This substitution with phenylalanine enhances the compound’s affinity for GnRH receptors, making it a more potent agonist . Additionally, the specific substitution can influence the duration and intensity of LH and FSH release .
Properties
IUPAC Name |
(2S)-N-[(2S)-1-[[(2S)-1-[[(2S)-1-[[(2S)-1-[[(2R)-1-[[(2S)-1-[[(2S)-1-[(2S)-2-[(2-amino-2-oxoethyl)carbamoyl]pyrrolidin-1-yl]-5-(diaminomethylideneamino)-1-oxopentan-2-yl]amino]-4-methyl-1-oxopentan-2-yl]amino]-1-oxo-3-phenylpropan-2-yl]amino]-3-(4-hydroxyphenyl)-1-oxopropan-2-yl]amino]-3-hydroxy-1-oxopropan-2-yl]amino]-3-(1H-indol-3-yl)-1-oxopropan-2-yl]amino]-3-(1H-imidazol-5-yl)-1-oxopropan-2-yl]-5-oxopyrrolidine-2-carboxamide | |
---|---|---|
Source | PubChem | |
URL | https://pubchem.ncbi.nlm.nih.gov | |
Description | Data deposited in or computed by PubChem | |
InChI |
InChI=1S/C62H81N17O13/c1-34(2)24-44(54(85)72-43(14-8-22-67-62(64)65)61(92)79-23-9-15-50(79)60(91)69-31-51(63)82)73-55(86)45(25-35-10-4-3-5-11-35)74-56(87)46(26-36-16-18-39(81)19-17-36)75-59(90)49(32-80)78-57(88)47(27-37-29-68-41-13-7-6-12-40(37)41)76-58(89)48(28-38-30-66-33-70-38)77-53(84)42-20-21-52(83)71-42/h3-7,10-13,16-19,29-30,33-34,42-50,68,80-81H,8-9,14-15,20-28,31-32H2,1-2H3,(H2,63,82)(H,66,70)(H,69,91)(H,71,83)(H,72,85)(H,73,86)(H,74,87)(H,75,90)(H,76,89)(H,77,84)(H,78,88)(H4,64,65,67)/t42-,43-,44-,45+,46-,47-,48-,49-,50-/m0/s1 | |
Source | PubChem | |
URL | https://pubchem.ncbi.nlm.nih.gov | |
Description | Data deposited in or computed by PubChem | |
InChI Key |
YQYQKOPVQDUQLX-HDJHSADSSA-N | |
Source | PubChem | |
URL | https://pubchem.ncbi.nlm.nih.gov | |
Description | Data deposited in or computed by PubChem | |
Canonical SMILES |
CC(C)CC(C(=O)NC(CCCN=C(N)N)C(=O)N1CCCC1C(=O)NCC(=O)N)NC(=O)C(CC2=CC=CC=C2)NC(=O)C(CC3=CC=C(C=C3)O)NC(=O)C(CO)NC(=O)C(CC4=CNC5=CC=CC=C54)NC(=O)C(CC6=CN=CN6)NC(=O)C7CCC(=O)N7 | |
Source | PubChem | |
URL | https://pubchem.ncbi.nlm.nih.gov | |
Description | Data deposited in or computed by PubChem | |
Isomeric SMILES |
CC(C)C[C@@H](C(=O)N[C@@H](CCCN=C(N)N)C(=O)N1CCC[C@H]1C(=O)NCC(=O)N)NC(=O)[C@@H](CC2=CC=CC=C2)NC(=O)[C@H](CC3=CC=C(C=C3)O)NC(=O)[C@H](CO)NC(=O)[C@H](CC4=CNC5=CC=CC=C54)NC(=O)[C@H](CC6=CN=CN6)NC(=O)[C@@H]7CCC(=O)N7 | |
Source | PubChem | |
URL | https://pubchem.ncbi.nlm.nih.gov | |
Description | Data deposited in or computed by PubChem | |
Molecular Formula |
C62H81N17O13 | |
Source | PubChem | |
URL | https://pubchem.ncbi.nlm.nih.gov | |
Description | Data deposited in or computed by PubChem | |
DSSTOX Substance ID |
DTXSID50206131 | |
Record name | LHRH, Phe(6)- | |
Source | EPA DSSTox | |
URL | https://comptox.epa.gov/dashboard/DTXSID50206131 | |
Description | DSSTox provides a high quality public chemistry resource for supporting improved predictive toxicology. | |
Molecular Weight |
1272.4 g/mol | |
Source | PubChem | |
URL | https://pubchem.ncbi.nlm.nih.gov | |
Description | Data deposited in or computed by PubChem | |
CAS No. |
57521-78-5 | |
Record name | LHRH, Phe(6)- | |
Source | ChemIDplus | |
URL | https://pubchem.ncbi.nlm.nih.gov/substance/?source=chemidplus&sourceid=0057521785 | |
Description | ChemIDplus is a free, web search system that provides access to the structure and nomenclature authority files used for the identification of chemical substances cited in National Library of Medicine (NLM) databases, including the TOXNET system. | |
Record name | LHRH, Phe(6)- | |
Source | EPA DSSTox | |
URL | https://comptox.epa.gov/dashboard/DTXSID50206131 | |
Description | DSSTox provides a high quality public chemistry resource for supporting improved predictive toxicology. | |
Retrosynthesis Analysis
AI-Powered Synthesis Planning: Our tool employs the Template_relevance Pistachio, Template_relevance Bkms_metabolic, Template_relevance Pistachio_ringbreaker, Template_relevance Reaxys, Template_relevance Reaxys_biocatalysis model, leveraging a vast database of chemical reactions to predict feasible synthetic routes.
One-Step Synthesis Focus: Specifically designed for one-step synthesis, it provides concise and direct routes for your target compounds, streamlining the synthesis process.
Accurate Predictions: Utilizing the extensive PISTACHIO, BKMS_METABOLIC, PISTACHIO_RINGBREAKER, REAXYS, REAXYS_BIOCATALYSIS database, our tool offers high-accuracy predictions, reflecting the latest in chemical research and data.
Strategy Settings
Precursor scoring | Relevance Heuristic |
---|---|
Min. plausibility | 0.01 |
Model | Template_relevance |
Template Set | Pistachio/Bkms_metabolic/Pistachio_ringbreaker/Reaxys/Reaxys_biocatalysis |
Top-N result to add to graph | 6 |
Feasible Synthetic Routes
Disclaimer and Information on In-Vitro Research Products
Please be aware that all articles and product information presented on BenchChem are intended solely for informational purposes. The products available for purchase on BenchChem are specifically designed for in-vitro studies, which are conducted outside of living organisms. In-vitro studies, derived from the Latin term "in glass," involve experiments performed in controlled laboratory settings using cells or tissues. It is important to note that these products are not categorized as medicines or drugs, and they have not received approval from the FDA for the prevention, treatment, or cure of any medical condition, ailment, or disease. We must emphasize that any form of bodily introduction of these products into humans or animals is strictly prohibited by law. It is essential to adhere to these guidelines to ensure compliance with legal and ethical standards in research and experimentation.