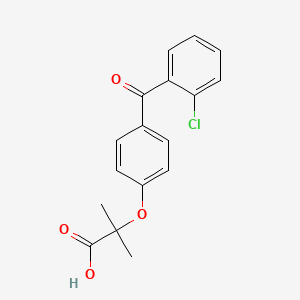
2-Chloro Fenofibric Acid
Overview
Description
2-Chloro Fenofibric Acid is a derivative of fenofibric acid, which is widely used in the treatment of hypercholesterolemia and hypertriglyceridemia. This compound is known for its lipid-regulating properties, making it a valuable agent in reducing cardiovascular disease risks .
Mechanism of Action
Target of Action
The primary target of 2-Chloro Fenofibric Acid, an active metabolite of the hyperlipidemic drug fenofibrate, is believed to be the peroxisome proliferator receptor alpha (PPAR-alpha) . This receptor plays a crucial role in the regulation of lipid metabolism. Additionally, it has been found to destabilize the receptor-binding domain (RBD) of the viral spike protein and therefore inhibit its binding to human angiotensin-converting enzyme 2 (hACE2) receptor .
Mode of Action
This compound activates PPAR-alpha, leading to a decrease in elevated low-density lipoprotein cholesterol, total cholesterol, triglycerides, and apolipoprotein B, while increasing high-density lipoprotein cholesterol . Furthermore, it has been shown to interact with a cryptic binding site on the RBD of the SARS-CoV-2 spike protein, altering the conformation of the binding loop of RBD and effectively reducing its binding affinity towards ACE2 .
Biochemical Pathways
The activation of PPAR-alpha by this compound stimulates lipoprotein lipase and inhibits apolipoprotein C-III, which in turn leads to lipolysis and a reduction in triglycerides . It also increases the expression of apolipoprotein A-I, A-II, and high-density lipoprotein .
Pharmacokinetics
Fenofibrate, from which this compound is derived, is absorbed from the gastrointestinal tract and is principally hydrolyzed by the CYP3A4 isozyme to its active and major metabolite, fenofibric acid . The absorption rate of fenofibrate is significantly affected by the fat content of ingested food .
Result of Action
The activation of PPAR-alpha by this compound leads to a decrease in elevated low-density lipoprotein cholesterol, total cholesterol, triglycerides, and apolipoprotein B, while increasing high-density lipoprotein cholesterol . This results in improved lipid profiles, which can be beneficial in the treatment of conditions such as hypercholesterolemia and hypertriglyceridemia . In the context of SARS-CoV-2, it has been shown to reduce viral infection by up to 70% in cultured cells .
Action Environment
The action of this compound can be influenced by various environmental factors. For instance, the absorption rate of fenofibrate, from which this compound is derived, is significantly affected by the fat content of ingested food This means that the bioavailability and efficacy of the compound can vary depending on dietary factors
Biochemical Analysis
Biochemical Properties
2-Chloro Fenofibric Acid, like its parent compound fenofibric acid, is expected to interact with various enzymes, proteins, and other biomolecules. Fenofibric acid is known to decrease elevated low-density lipoprotein cholesterol, total cholesterol, triglycerides, apolipoprotein B, while increasing high-density lipoprotein cholesterol . It is reasonable to expect that this compound may have similar interactions and effects.
Cellular Effects
Fenofibric acid, the parent compound, is known to influence cell function by altering lipid metabolism . It can impact cell signaling pathways, gene expression, and cellular metabolism .
Molecular Mechanism
Fenofibric acid, the parent compound, is known to exert its effects at the molecular level by binding to peroxisome proliferator-activated receptor alpha (PPARα), which leads to increased lipolysis and reduction of apolipoprotein C-III .
Temporal Effects in Laboratory Settings
The temporal effects of this compound in laboratory settings are not well-studied. Fenofibric acid has been studied extensively. It has been found that fenofibric acid does not accumulate significantly in plasma or tissues due to its short half-life .
Dosage Effects in Animal Models
The effects of different dosages of this compound in animal models have not been reported. Studies on fenofibrate, a prodrug of fenofibric acid, have shown that the incidence of liver enzyme elevations increases with dosage .
Metabolic Pathways
Fenofibric acid is predominantly conjugated with glucuronic acid and then excreted in urine .
Transport and Distribution
Fenofibric acid is known to be extensively absorbed only in the presence of food and is transported through the bloodstream by albumin .
Subcellular Localization
Fenofibric acid, the parent compound, is known to be taken up by both the liver and kidney .
Preparation Methods
Synthetic Routes and Reaction Conditions: The synthesis of 2-Chloro Fenofibric Acid typically involves the chlorination of fenofibric acid. One common method includes dissolving 4’-chloro-4-hydroxy benzophenone in acetone, adding sodium hydroxide, and heating the mixture. Chloroform is then added, and the reaction mixture is heated to reflux. The product is isolated by adjusting the pH and extracting with dichloromethane .
Industrial Production Methods: Industrial production of this compound follows similar synthetic routes but on a larger scale. The process involves precise control of reaction conditions to ensure high yield and purity. The use of continuous flow reactors and automated systems helps in scaling up the production efficiently .
Chemical Reactions Analysis
Types of Reactions: 2-Chloro Fenofibric Acid undergoes various chemical reactions, including:
Oxidation: This reaction can be carried out using oxidizing agents like potassium permanganate.
Reduction: Reduction reactions typically involve reagents such as lithium aluminum hydride.
Substitution: Halogenation and other substitution reactions are common, using reagents like chlorine or bromine.
Common Reagents and Conditions:
Oxidation: Potassium permanganate in an acidic medium.
Reduction: Lithium aluminum hydride in anhydrous ether.
Substitution: Chlorine gas or bromine in the presence of a catalyst.
Major Products: The major products formed from these reactions include various chlorinated derivatives and reduced forms of the compound, depending on the specific reaction conditions .
Scientific Research Applications
2-Chloro Fenofibric Acid has several applications in scientific research:
Comparison with Similar Compounds
Fenofibric Acid: The parent compound, widely used for its lipid-regulating properties.
Clofibrate: Another fibrate used to lower lipid levels.
Gemfibrozil: A fibrate that also targets lipid metabolism but has a different chemical structure.
Uniqueness: 2-Chloro Fenofibric Acid is unique due to its chlorinated structure, which may confer different pharmacokinetic properties and potentially enhanced lipid-lowering effects compared to its non-chlorinated counterparts .
Properties
IUPAC Name |
2-[4-(2-chlorobenzoyl)phenoxy]-2-methylpropanoic acid | |
---|---|---|
Source | PubChem | |
URL | https://pubchem.ncbi.nlm.nih.gov | |
Description | Data deposited in or computed by PubChem | |
InChI |
InChI=1S/C17H15ClO4/c1-17(2,16(20)21)22-12-9-7-11(8-10-12)15(19)13-5-3-4-6-14(13)18/h3-10H,1-2H3,(H,20,21) | |
Source | PubChem | |
URL | https://pubchem.ncbi.nlm.nih.gov | |
Description | Data deposited in or computed by PubChem | |
InChI Key |
OTLZAXXVHHHGDU-UHFFFAOYSA-N | |
Source | PubChem | |
URL | https://pubchem.ncbi.nlm.nih.gov | |
Description | Data deposited in or computed by PubChem | |
Canonical SMILES |
CC(C)(C(=O)O)OC1=CC=C(C=C1)C(=O)C2=CC=CC=C2Cl | |
Source | PubChem | |
URL | https://pubchem.ncbi.nlm.nih.gov | |
Description | Data deposited in or computed by PubChem | |
Molecular Formula |
C17H15ClO4 | |
Source | PubChem | |
URL | https://pubchem.ncbi.nlm.nih.gov | |
Description | Data deposited in or computed by PubChem | |
DSSTOX Substance ID |
DTXSID90614897 | |
Record name | 2-[4-(2-Chlorobenzoyl)phenoxy]-2-methylpropanoic acid | |
Source | EPA DSSTox | |
URL | https://comptox.epa.gov/dashboard/DTXSID90614897 | |
Description | DSSTox provides a high quality public chemistry resource for supporting improved predictive toxicology. | |
Molecular Weight |
318.7 g/mol | |
Source | PubChem | |
URL | https://pubchem.ncbi.nlm.nih.gov | |
Description | Data deposited in or computed by PubChem | |
CAS No. |
61024-31-5 | |
Record name | 2-[4-(2-Chlorobenzoyl)phenoxy]-2-methylpropanoic acid | |
Source | EPA DSSTox | |
URL | https://comptox.epa.gov/dashboard/DTXSID90614897 | |
Description | DSSTox provides a high quality public chemistry resource for supporting improved predictive toxicology. | |
Retrosynthesis Analysis
AI-Powered Synthesis Planning: Our tool employs the Template_relevance Pistachio, Template_relevance Bkms_metabolic, Template_relevance Pistachio_ringbreaker, Template_relevance Reaxys, Template_relevance Reaxys_biocatalysis model, leveraging a vast database of chemical reactions to predict feasible synthetic routes.
One-Step Synthesis Focus: Specifically designed for one-step synthesis, it provides concise and direct routes for your target compounds, streamlining the synthesis process.
Accurate Predictions: Utilizing the extensive PISTACHIO, BKMS_METABOLIC, PISTACHIO_RINGBREAKER, REAXYS, REAXYS_BIOCATALYSIS database, our tool offers high-accuracy predictions, reflecting the latest in chemical research and data.
Strategy Settings
Precursor scoring | Relevance Heuristic |
---|---|
Min. plausibility | 0.01 |
Model | Template_relevance |
Template Set | Pistachio/Bkms_metabolic/Pistachio_ringbreaker/Reaxys/Reaxys_biocatalysis |
Top-N result to add to graph | 6 |
Feasible Synthetic Routes
Disclaimer and Information on In-Vitro Research Products
Please be aware that all articles and product information presented on BenchChem are intended solely for informational purposes. The products available for purchase on BenchChem are specifically designed for in-vitro studies, which are conducted outside of living organisms. In-vitro studies, derived from the Latin term "in glass," involve experiments performed in controlled laboratory settings using cells or tissues. It is important to note that these products are not categorized as medicines or drugs, and they have not received approval from the FDA for the prevention, treatment, or cure of any medical condition, ailment, or disease. We must emphasize that any form of bodily introduction of these products into humans or animals is strictly prohibited by law. It is essential to adhere to these guidelines to ensure compliance with legal and ethical standards in research and experimentation.