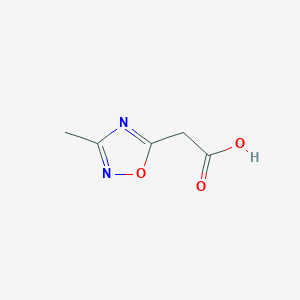
2-(3-Methyl-1,2,4-oxadiazol-5-yl)acetic acid
Overview
Description
2-(3-Methyl-1,2,4-oxadiazol-5-yl)acetic acid is an organic compound featuring a five-membered heterocyclic ring containing two nitrogen atoms and one oxygen atom. This compound is known for its versatile applications in various fields, including medicinal chemistry, material science, and agrochemicals. Its unique structure allows it to participate in a wide range of chemical reactions, making it a valuable building block in synthetic chemistry.
Preparation Methods
Synthesis via Amidoximes and Maleates/Fumarates in Basic Medium
A prominent method involves the reaction of amidoximes with maleates or fumarates in a basic medium such as sodium hydroxide in dimethyl sulfoxide (DMSO). This approach facilitates both O-acylation and 1,2,4-oxadiazole ring closure at room temperature, yielding 1,2,4-oxadiazin-5-ones and related acids.
- Amidoxime (2 mmol) and ester (4 mmol) are dissolved in DMSO (3 mL).
- Powdered NaOH (240 mg, 4 mmol) is added rapidly.
- The mixture is stirred at room temperature for 18 hours.
- The reaction is quenched with cold brine and washed with toluene.
- Acidification with hydrochloric acid to pH ~1 precipitates the product.
- The solid is filtered, washed, and dried at 50 °C.
- Initial yields of the acid product were around 37%, improved to 65% by optimizing reagent ratios and reaction time.
- NaOH was found to be the most effective base for acid formation, while tert-butoxide sodium (t-BuONa) favored ester formation.
- Prolonged reaction times or excess reagents led to side products such as 4-methylbenzonitrile.
- The method tolerates various substituents on the amidoxime, including heterocyclic groups.
Entry | Base | Ester Amount (mmol) | Reaction Time (h) | Yield of Acid (%) | Notes |
---|---|---|---|---|---|
1 | NaOH | 4 | 18 | 37 | Initial trial |
2 | NaOH | 4 | 18 | 65 | Optimized conditions |
3 | NaOH | >4 | >18 | <65 | More side products formed |
4 | t-BuONa | 4 | 18 | - | Ester product favored |
Table 1: Optimization of Reaction Conditions for Acid Formation via Amidoximes and Maleates
Condensation of p-Tolyl Amidoxime with Methyl 2-Haloacetates
Another effective route uses the condensation of p-tolyl amidoxime with methyl 2-haloacetates (e.g., methyl 2-chloroacetate) in the presence of bases such as NaOH or potassium hydroxide (KOH).
- The reaction is performed in DMSO at room temperature.
- Use of methyl 2-chloroacetate improved yields compared to other esters.
- NaOH and KOH gave comparable yields (~35-38%), while t-BuONa favored different products.
- Typical reaction time is 18 hours with stirring at room temperature.
- Amidoxime (2 mmol) is dissolved in DMSO (3 mL).
- Base (4 mmol) is added rapidly.
- After 10 minutes, methyl 2-haloacetate (2.4 mmol) is added.
- The mixture is stirred for 18 hours at room temperature.
- Acidification with aqueous HCl precipitates the product, which is filtered and dried.
Entry | Base | Haloacetate | Yield (%) | Notes |
---|---|---|---|---|
1 | NaOH | Methyl 2-chloroacetate | 35 | Initial trial |
2 | KOH | Methyl 2-chloroacetate | 38 | Slightly improved yield |
3 | t-BuONa | Methyl 2-chloroacetate | - | Different product formation |
Table 2: Reaction Outcomes for Amidoxime and Methyl 2-Haloacetates Condensation
Multi-Step Synthesis Involving Carbonyldiimidazole and Hydrazides (Patent Method)
A more complex synthetic route described in patent literature involves:
- Activation of a protected piperidyl-substituted acetic acid with 1,1′-carbonyldiimidazole in tetrahydrofuran (THF).
- Subsequent reaction with acetohydrazide to form hydrazide intermediates.
- Multiple extraction and purification steps including acid-base extractions and solvent partitioning.
- Final condensation steps with aldehyde derivatives and reductive amination.
Although this method is more elaborate and designed for pharmaceutical intermediates, it demonstrates the versatility of oxadiazole acetic acid derivatives synthesis under controlled conditions, including temperature regulation (5–47 °C) and careful pH adjustments.
Mechanistic Insights and Reaction Pathways
- Amidoximes deprotonate in basic media and nucleophilically attack activated double bonds of maleates or fumarates.
- Intermediate adducts undergo intramolecular cyclization via amino group attack on alkoxycarbonyl groups.
- Protonation and methanol elimination finalize the formation of the oxadiazole ring.
- The base choice influences whether acids or esters predominate.
Summary Table of Preparation Methods
Method | Key Reactants | Base Used | Solvent | Temp. | Yield Range (%) | Notes |
---|---|---|---|---|---|---|
Amidoximes + Maleates/Fumarates | Amidoximes, Dimethyl maleate | NaOH, t-BuONa | DMSO | Room temp. | 37–65 | NaOH favors acids; t-BuONa favors esters |
Amidoximes + Methyl 2-Haloacetates | p-Tolyl amidoxime, methyl 2-chloroacetate | NaOH, KOH, t-BuONa | DMSO | Room temp. | 35–42 | Methyl 2-chloroacetate improves yield |
Multi-step Carbonyldiimidazole Route | Protected acetic acid, hydrazides | Various | THF | 5–47 °C | Not specified | Complex, pharmaceutical intermediate synthesis |
Chemical Reactions Analysis
Types of Reactions: 2-(3-Methyl-1,2,4-oxadiazol-5-yl)acetic acid undergoes various chemical reactions, including:
Oxidation: The compound can be oxidized to form corresponding oxadiazole derivatives.
Reduction: Reduction reactions can convert the oxadiazole ring into other heterocyclic structures.
Substitution: The acetic acid moiety allows for substitution reactions, introducing different functional groups.
Common Reagents and Conditions:
Oxidation: Common oxidizing agents include potassium permanganate and hydrogen peroxide.
Reduction: Reducing agents such as lithium aluminum hydride or sodium borohydride are often used.
Substitution: Reagents like alkyl halides or acyl chlorides can be used under basic or acidic conditions.
Major Products Formed: The reactions typically yield a variety of oxadiazole derivatives, which can be further functionalized for specific applications .
Scientific Research Applications
Pharmaceutical Applications
1.1 Neuropharmacology
Recent studies have highlighted the potential of 2-(3-Methyl-1,2,4-oxadiazol-5-yl)acetic acid as a pharmacological agent in the treatment of neurodegenerative diseases such as Alzheimer's disease and tauopathies. The compound is being investigated for its ability to modulate tau protein aggregation, which is a hallmark of these conditions. Research indicates that compounds similar to this compound can inhibit the oligomerization of tau proteins, thereby potentially reducing neurofibrillary tangles associated with Alzheimer's disease .
1.2 Kappa Opioid Receptor Antagonism
Another significant application of this compound is in the development of selective Kappa Opioid Receptor (KOR) antagonists. Studies have shown that derivatives of this compound exhibit potent activity at the KOR, which is implicated in various neuropsychiatric disorders. For instance, a related compound demonstrated efficacy in preclinical models for treating depression and substance abuse disorders .
Toxicology and Safety
Safety data for this compound indicate that while it is intended for laboratory use only, standard precautions should be taken to avoid inhalation or skin contact. The compound does not currently have extensive toxicological data available; however, general safety measures include using personal protective equipment (PPE) when handling the substance .
Case Studies and Research Findings
Case Study: Neuroprotective Effects
A study published in Journal of Neurochemistry explored the neuroprotective effects of a derivative related to this compound in a mouse model of Alzheimer's disease. The results indicated that treatment with the compound led to a significant reduction in tau pathology and improved cognitive function compared to control groups .
Case Study: KOR Antagonism and Depression Treatment
In another study focusing on KOR antagonism, researchers evaluated a series of compounds based on the oxadiazole structure for their effects on depression-like behaviors in rodent models. The findings suggested that certain derivatives exhibited significant antidepressant-like effects and could represent a novel therapeutic approach for treatment-resistant depression .
Mechanism of Action
The mechanism of action of 2-(3-Methyl-1,2,4-oxadiazol-5-yl)acetic acid involves its interaction with specific molecular targets. The oxadiazole ring can act as a hydrogen bond acceptor, facilitating interactions with enzymes and receptors. This interaction can modulate biological pathways, leading to the compound’s observed effects. For instance, in medicinal applications, it may inhibit the activity of certain enzymes, thereby exerting its therapeutic effects .
Comparison with Similar Compounds
1,2,4-Oxadiazole-5-acetic acid: Similar structure but without the methyl group.
3-Methyl-1,2,4-oxadiazole: Lacks the acetic acid moiety.
2-(3-Methyl-1,2,4-oxadiazol-5-yl)-phenoxy-acetic acid: Contains a phenoxy group instead of a simple acetic acid moiety.
Uniqueness: 2-(3-Methyl-1,2,4-oxadiazol-5-yl)acetic acid is unique due to its combination of the oxadiazole ring and the acetic acid moiety, which provides a balance of reactivity and stability. This makes it a versatile intermediate for various synthetic applications, distinguishing it from other similar compounds .
Biological Activity
2-(3-Methyl-1,2,4-oxadiazol-5-yl)acetic acid is a compound that has garnered attention due to its diverse biological activities. This article explores its pharmacological properties, mechanisms of action, and potential therapeutic applications based on recent research findings.
Chemical Structure and Properties
The compound features a 1,2,4-oxadiazole ring, which is known for its biological significance. The presence of the acetic acid moiety enhances its solubility and potential bioactivity.
Anticancer Activity
Recent studies have highlighted the anticancer potential of oxadiazole derivatives. For instance, compounds similar to this compound have shown promising results against various cancer cell lines. A study reported that certain oxadiazole derivatives exhibited significant cytotoxicity against HepG2 liver cancer cells with IC50 values ranging from 4.2 to 7.29 µM, indicating their potential as effective anticancer agents .
Antimicrobial Activity
The compound has also been evaluated for its antimicrobial properties. It demonstrated moderate to good antibacterial activity against both Gram-positive and Gram-negative bacteria. Minimum inhibitory concentration (MIC) values ranged from 4.69 to 22.9 µM against various bacterial strains such as Bacillus subtilis and Staphylococcus aureus. Furthermore, antifungal activity was observed with MIC values against Candida albicans ranging from 16.69 to 78.23 µM .
The mechanisms underlying the biological activity of this compound involve several pathways:
- Cell Cycle Disruption : Some studies suggest that oxadiazole derivatives can induce cell cycle arrest in cancer cells, leading to apoptosis.
- Inhibition of Enzymatic Activity : The compound may inhibit specific enzymes involved in cancer progression or microbial metabolism.
- Modulation of Signaling Pathways : Research indicates that these compounds can affect key signaling pathways related to cell proliferation and survival.
Study on Anticancer Effects
In a recent study focusing on the anticancer effects of oxadiazole derivatives, researchers synthesized several analogs and tested their efficacy against HepG2 cells. The study found that three specific compounds exhibited significantly higher toxicity towards cancer cells compared to normal cells, suggesting their potential as targeted therapies .
Compound | IC50 (µM) | Selectivity Ratio |
---|---|---|
Compound A | 5.5 | 10 |
Compound B | 7.29 | 8.7 |
Compound C | 4.2 | 41 |
Study on Antimicrobial Activity
Another study evaluated the antimicrobial properties of various oxadiazole derivatives including this compound. The results indicated effective inhibition of bacterial growth across multiple strains:
Bacterial Strain | MIC (µM) |
---|---|
Bacillus subtilis | 4.69 |
Staphylococcus aureus | 5.64 |
Escherichia coli | 8.33 |
Pseudomonas aeruginosa | 13.40 |
Q & A
Basic Research Questions
Q. What are the standard synthetic routes for 2-(3-Methyl-1,2,4-oxadiazol-5-yl)acetic acid?
The compound is typically synthesized via intramolecular cyclization of hydrazide intermediates. For example, sodium salts of heterocyclic precursors (e.g., xanthine derivatives) can react with ethyl isothiocyanate, followed by cyclization to form the oxadiazole core. Subsequent alkylation with chloroacetic acid introduces the acetic acid moiety . Alternative methods include continuous-flow synthesis, which enhances reaction efficiency and sustainability by optimizing temperature, solvent, and nucleophile selection .
Q. What analytical techniques confirm the structure and purity of this compound?
Characterization involves elemental analysis (to verify stoichiometry), IR spectroscopy (to identify functional groups like C=O and N-O), ¹H NMR (to resolve proton environments), and mass spectrometry (to confirm molecular weight). Chromatographic methods (e.g., TLC or HPLC) assess purity, while X-ray crystallography may resolve crystal structures for salts or derivatives .
Q. How are purification and solubility challenges addressed?
Recrystallization from polar aprotic solvents (e.g., DMF/acetic acid mixtures) is commonly used. Salts of the compound (e.g., sodium, potassium, or transition-metal complexes) improve solubility in aqueous media, enabling pharmacological testing .
Advanced Research Questions
Q. How can reaction selectivity be optimized during alkylation or cyclization steps?
Screening bases (e.g., NaOAc), solvents (e.g., acetic acid), and nucleophiles under varying temperatures can influence regioselectivity. For example, continuous-flow systems reduce side reactions by ensuring precise control over reaction parameters . Contradictions in yield or selectivity between studies often arise from differences in reactant stoichiometry or catalyst choice, necessitating mechanistic studies (e.g., DFT calculations) .
Q. What strategies resolve discrepancies in pharmacological activity data for derivatives?
Structural modifications, such as introducing hydroxy(phenyl)methyl groups or forming metal complexes, alter bioactivity. For instance, zinc or copper salts may enhance antimicrobial properties, while organic base salts (e.g., morpholine) improve bioavailability. Comparative studies using standardized assays (e.g., MIC for antimicrobial activity) are critical to validate results .
Q. How can computational methods guide the design of novel derivatives?
Molecular docking and QSAR models predict binding affinities to target enzymes (e.g., bacterial dihydrofolate reductase). For example, substituents on the oxadiazole ring can be tailored to optimize hydrogen bonding or hydrophobic interactions, as demonstrated in triazole-thiadiazole hybrids .
Q. Methodological Considerations
- Salt Synthesis : Reacting the free acid with hydroxides (NaOH/KOH) or metal sulfates (e.g., CuSO₄) in ethanol yields salts. Excess base or metal ions must be avoided to prevent precipitation of hydroxides .
- Stability Testing : Accelerated degradation studies (e.g., under UV light or high humidity) assess shelf life. IR and NMR track structural integrity over time .
Properties
IUPAC Name |
2-(3-methyl-1,2,4-oxadiazol-5-yl)acetic acid | |
---|---|---|
Source | PubChem | |
URL | https://pubchem.ncbi.nlm.nih.gov | |
Description | Data deposited in or computed by PubChem | |
InChI |
InChI=1S/C5H6N2O3/c1-3-6-4(10-7-3)2-5(8)9/h2H2,1H3,(H,8,9) | |
Source | PubChem | |
URL | https://pubchem.ncbi.nlm.nih.gov | |
Description | Data deposited in or computed by PubChem | |
InChI Key |
SPLXTCXODDVKKW-UHFFFAOYSA-N | |
Source | PubChem | |
URL | https://pubchem.ncbi.nlm.nih.gov | |
Description | Data deposited in or computed by PubChem | |
Canonical SMILES |
CC1=NOC(=N1)CC(=O)O | |
Source | PubChem | |
URL | https://pubchem.ncbi.nlm.nih.gov | |
Description | Data deposited in or computed by PubChem | |
Molecular Formula |
C5H6N2O3 | |
Source | PubChem | |
URL | https://pubchem.ncbi.nlm.nih.gov | |
Description | Data deposited in or computed by PubChem | |
DSSTOX Substance ID |
DTXSID20612220 | |
Record name | (3-Methyl-1,2,4-oxadiazol-5-yl)acetic acid | |
Source | EPA DSSTox | |
URL | https://comptox.epa.gov/dashboard/DTXSID20612220 | |
Description | DSSTox provides a high quality public chemistry resource for supporting improved predictive toxicology. | |
Molecular Weight |
142.11 g/mol | |
Source | PubChem | |
URL | https://pubchem.ncbi.nlm.nih.gov | |
Description | Data deposited in or computed by PubChem | |
CAS No. |
55151-91-2 | |
Record name | (3-Methyl-1,2,4-oxadiazol-5-yl)acetic acid | |
Source | EPA DSSTox | |
URL | https://comptox.epa.gov/dashboard/DTXSID20612220 | |
Description | DSSTox provides a high quality public chemistry resource for supporting improved predictive toxicology. | |
Synthesis routes and methods
Procedure details
Retrosynthesis Analysis
AI-Powered Synthesis Planning: Our tool employs the Template_relevance Pistachio, Template_relevance Bkms_metabolic, Template_relevance Pistachio_ringbreaker, Template_relevance Reaxys, Template_relevance Reaxys_biocatalysis model, leveraging a vast database of chemical reactions to predict feasible synthetic routes.
One-Step Synthesis Focus: Specifically designed for one-step synthesis, it provides concise and direct routes for your target compounds, streamlining the synthesis process.
Accurate Predictions: Utilizing the extensive PISTACHIO, BKMS_METABOLIC, PISTACHIO_RINGBREAKER, REAXYS, REAXYS_BIOCATALYSIS database, our tool offers high-accuracy predictions, reflecting the latest in chemical research and data.
Strategy Settings
Precursor scoring | Relevance Heuristic |
---|---|
Min. plausibility | 0.01 |
Model | Template_relevance |
Template Set | Pistachio/Bkms_metabolic/Pistachio_ringbreaker/Reaxys/Reaxys_biocatalysis |
Top-N result to add to graph | 6 |
Feasible Synthetic Routes
Disclaimer and Information on In-Vitro Research Products
Please be aware that all articles and product information presented on BenchChem are intended solely for informational purposes. The products available for purchase on BenchChem are specifically designed for in-vitro studies, which are conducted outside of living organisms. In-vitro studies, derived from the Latin term "in glass," involve experiments performed in controlled laboratory settings using cells or tissues. It is important to note that these products are not categorized as medicines or drugs, and they have not received approval from the FDA for the prevention, treatment, or cure of any medical condition, ailment, or disease. We must emphasize that any form of bodily introduction of these products into humans or animals is strictly prohibited by law. It is essential to adhere to these guidelines to ensure compliance with legal and ethical standards in research and experimentation.