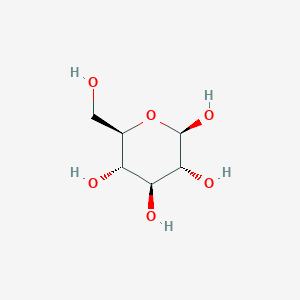
beta-D-Glucose
Overview
Description
Beta-D-glucose (C₆H₁₂O₆) is a monosaccharide and a fundamental carbohydrate in biological systems. Structurally, it exists predominantly in a six-membered pyranose ring conformation, where the hydroxyl (-OH) group at the anomeric carbon (C1) is positioned above the ring plane, distinguishing it from its alpha anomer . In aqueous solutions, this compound constitutes ~64% of the equilibrium mixture (with ~36% alpha-D-glucose and <0.01% linear form), driven by spontaneous mutarotation .
Mechanism of Action
Target of Action
Beta-D-Glucose, a naturally occurring compound found in fruits and other parts of plants , interacts with various targets in living organisms. It is known to interact with several enzymes such as DNA, Chondroitinase-B, Endoglucanase 5A, Lactase-like protein, Glycogen phosphorylase, Xylose isomerase, Glucokinase, Ferrichrome-iron receptor, and Hexokinase-1 . These enzymes play crucial roles in various biochemical processes, including energy production, metabolism, and cellular function .
Mode of Action
This compound’s mode of action is primarily through its interaction with these enzymes. For instance, Glucokinase, an enzyme found in the liver and beta cells of the pancreas, catalyzes the initial step in the utilization of glucose at physiological glucose concentration . This compound is also known to interact with Hexokinase-1, another key enzyme involved in the glycolytic pathway . The interaction of this compound with these enzymes leads to various biochemical changes within the cell .
Biochemical Pathways
This compound plays a significant role in the glycolytic pathway, also known as the Meyerhoff, Embden, Parnas pathway . This pathway involves the anaerobic conversion of glucose to pyruvate, providing the cell with ATP under anaerobic conditions and supplying precursors for the biosynthesis of proteins, lipids, nucleic acids, and polysaccharides . The enzymes involved in glycolysis, including Hexokinase and Phosphofructokinase 1 (PFK 1), are regulated by small molecules, and this compound plays a crucial role in this regulation .
Pharmacokinetics
While specific pharmacokinetic data on this compound is limited, it is known that the compound exhibits dose-proportional pharmacokinetics without apparent accumulation in the body . This suggests that this compound is metabolized and excreted efficiently, impacting its bioavailability.
Result of Action
The primary result of this compound’s action is the provision of energy for living organisms . By participating in glycolysis, this compound contributes to the production of ATP, the primary energy currency of the cell . Additionally, the interaction of this compound with various enzymes can lead to changes in cellular function and metabolism .
Action Environment
The action of this compound is influenced by various environmental factors. For instance, the activity of this compound-degrading enzymes can be affected by factors such as temperature and pH . Additionally, the presence of other molecules can impact the action of this compound. For example, in the presence of certain microbial structures, plant Beta-glucanases can hydrolyze Beta-glucans, triggering the activation of global responses .
Biochemical Analysis
Biochemical Properties
Beta-D-Glucose plays a crucial role in various biochemical reactions. It is the primary source of energy for cells and is metabolized through glycolysis. This compound interacts with various enzymes, such as hexokinase and glucokinase, which phosphorylate glucose to glucose-6-phosphate, the first step in most glucose metabolism pathways .
Cellular Effects
This compound impacts various types of cells and cellular processes. It influences cell function by providing the energy needed for cellular processes. It also impacts cell signaling pathways, gene expression, and cellular metabolism. For instance, high levels of this compound can lead to increased insulin production in beta cells of the pancreas .
Molecular Mechanism
The molecular mechanism of this compound involves its conversion to glucose-6-phosphate by the action of hexokinase or glucokinase. This is a crucial step as it keeps glucose inside the cell and makes it available for various metabolic pathways .
Temporal Effects in Laboratory Settings
In laboratory settings, this compound shows stability over time. Its effects on cellular function can vary depending on the concentration and duration of exposure .
Dosage Effects in Animal Models
In animal models, the effects of this compound can vary with different dosages. High doses of glucose can lead to hyperglycemia and insulin resistance, while low doses may not provide sufficient energy for normal cellular function .
Metabolic Pathways
This compound is involved in several metabolic pathways, including glycolysis, gluconeogenesis, and the pentose phosphate pathway. It interacts with various enzymes in these pathways, such as hexokinase, phosphofructokinase, and glucose-6-phosphate dehydrogenase .
Transport and Distribution
This compound is transported into cells via glucose transporters (GLUTs). Once inside the cell, it is phosphorylated to glucose-6-phosphate to prevent it from leaving the cell .
Subcellular Localization
Inside the cell, this compound is primarily found in the cytoplasm where glycolysis takes place. It can also be found in the mitochondria where it can be further metabolized to produce ATP .
Biological Activity
Beta-D-glucose, a simple sugar and a fundamental building block of carbohydrates, plays a significant role in various biological processes. This article explores its biological activity, including its immunomodulatory effects, antioxidant properties, and potential applications in cancer treatment.
Structure and Sources
This compound is a monosaccharide that is crucial for energy metabolism in living organisms. It is primarily found in fruits, vegetables, and as a component of polysaccharides such as cellulose and starch. The structure of this compound allows it to participate in numerous biochemical reactions, making it vital for cellular functions.
Immunomodulatory Effects
This compound has been identified as a biological response modulator (BRM), influencing immune system activity. Research indicates that it can enhance the function of various immune cells, including macrophages and natural killer (NK) cells. These effects are mediated through the activation of specific receptors such as Dectin-1 and complement receptors, which trigger immune responses against pathogens .
Key Findings:
- Macrophage Activation : this compound promotes the production of cytokines such as interleukin-1 (IL-1) and tumor necrosis factor-alpha (TNF-α), enhancing the body's ability to combat infections .
- Dendritic Cell Maturation : It facilitates the maturation of dendritic cells, thereby improving antigen presentation and adaptive immune responses .
- Cancer Immunotherapy : this compound derivatives like (1→3)-β-glucan are being explored for their potential in cancer immunotherapy due to their ability to stimulate immune responses against tumors .
Antioxidant Properties
This compound exhibits antioxidant activity, which helps protect cells from oxidative stress. This property is particularly important in preventing chronic diseases associated with oxidative damage, including cancer and cardiovascular diseases.
Research Highlights:
- Scavenging Free Radicals : Studies have shown that this compound can scavenge free radicals, thereby reducing oxidative stress in cells .
- Cellular Protection : In vitro experiments demonstrate that this compound protects cellular components from damage caused by reactive oxygen species (ROS) .
Anti-Cancer Activity
Recent studies have highlighted the anti-cancer potential of this compound and its derivatives. For instance, 1,2,3,4,6-penta-O-galloyl-beta-D-glucose has shown significant inhibitory effects on various cancer cell lines.
Case Studies:
- Prostate Cancer : In vivo studies indicated that this compound can inhibit tumor growth through mechanisms involving apoptosis and cell cycle arrest .
- Breast Cancer : Research has demonstrated that this compound derivatives can induce apoptosis in breast cancer cells by activating pro-apoptotic pathways .
Data Summary
The following table summarizes key biological activities associated with this compound:
Scientific Research Applications
Biochemical Applications
Energy Source
Beta-D-glucose serves as a primary energy source for living organisms. It is involved in metabolic pathways such as glycolysis and the citric acid cycle, where it is broken down to produce ATP, the energy currency of cells .
Structural Role
In addition to its role as an energy source, this compound is a building block for polysaccharides like cellulose and glycogen. These polysaccharides are vital for energy storage and structural integrity in plants and animals .
Pharmaceutical Applications
Glucose Oxidase Production
this compound is utilized in the production of glucose oxidase (GOx), an enzyme that catalyzes the oxidation of glucose to gluconic acid while producing hydrogen peroxide. This enzyme has applications in biosensors for glucose monitoring, which are essential for diabetes management . Recent advancements have highlighted its use in developing novel biosensors that enhance the accuracy of glucose measurements.
Antimicrobial Properties
Research indicates that this compound derivatives can enhance the antibacterial activity of certain compounds. For instance, studies have shown that extracts containing this compound can inhibit methicillin-resistant Staphylococcus aureus (MRSA) through synergistic effects with antibiotics .
Food Industry Applications
Sweetening Agent
this compound is commonly used as a sweetener in various food products due to its sweetness profile and ability to enhance flavor. It is also involved in Maillard reactions, contributing to the browning and flavor development in baked goods.
Preservative Role
In food preservation, this compound can act as a stabilizer and preservative by inhibiting microbial growth through its metabolic pathways. This application is particularly valuable in extending the shelf life of food products .
Clinical Applications
Diabetes Research
this compound plays a significant role in diabetes research, particularly in understanding glucose metabolism and insulin response. Studies have explored its effects on pancreatic beta-cell function, indicating potential therapeutic strategies for diabetes management by improving glucose homeostasis .
Case Study: Stem Cell Therapy for Diabetes
Recent clinical trials have investigated the use of encapsulated stem cell-derived beta cells to improve glucose control in patients with Type 1 Diabetes (T1D). The results showed that some patients experienced improved glycemic control post-implantation, highlighting the potential of this compound in regenerative medicine .
Table 1: Summary of Applications of this compound
Q & A
Basic Research Questions
Q. How can researchers experimentally distinguish α-D-glucose from β-D-glucose isomers?
Methodological Answer :
- Enzymatic specificity : Use β-D-glucose oxidase, which selectively oxidizes the β-anomer to D-glucono-1,5-lactone and H₂O₂, enabling spectrophotometric detection .
- NMR spectroscopy : The C1 carbon of β-D-glucose resonates at ~97 ppm (vs. ~93 ppm for α-D-glucose) in D₂O, confirmed by ¹³C NMR .
- Polarimetry : Monitor mutarotation kinetics (e.g., α → β equilibrium) at 589 nm, with final optical rotation of +52.7° for β-D-glucose .
Q. What analytical methods are optimal for quantifying β-D-glucose in biological samples?
Methodological Answer :
- HPLC-RI/MS : Separate anomers using a NH₂-bonded column (acetonitrile/water mobile phase) with refractive index or mass spectrometry detection .
- Enzymatic assays : Couple β-D-glucose oxidase with peroxidase and chromogenic substrates (e.g., o-dianisidine) for colorimetric quantification .
- Biosensors : Amperometric electrodes functionalized with glucose oxidase enable real-time monitoring in cell cultures .
Q. What role does β-D-glucose play in glycogen synthesis?
Methodological Answer : β-D-glucose is phosphorylated to β-D-glucose 6-phosphate (G6P) by hexokinase. Phosphoglucomutase isomerizes β-D-G6P to α-D-G6P, the substrate for glycogen synthase . Isotopic tracing (¹³C-glucose) with LC-MS/MS quantifies flux into glycogen .
Advanced Research Questions
Q. How does β-D-glucose oxidase specificity impact enzymatic assay design?
Methodological Answer :
- Anomer equilibration : Pre-incubate samples with mutarotase (aldose 1-epimerase) to ensure α→β conversion, avoiding underestimation of total glucose .
- Kinetic validation : Compare reaction rates using purified α- and β-anomers. Contradictions in literature values often stem from incomplete equilibration .
- Cofactor optimization : Saturate assays with O₂ (for oxidase) or NAD⁺ (for dehydrogenase) to match enzyme-specific requirements .
Q. What synthetic challenges arise in preparing β-D-glucose derivatives for glycobiology?
Methodological Answer :
- Protecting groups : Acetylation (e.g., pentaacetate synthesis via acetic anhydride/pyridine) stabilizes the β-configuration during glycosylation .
- Catalyst optimization : Lewis acids like BF₃·Et₂O improve glycosylation efficiency but require anhydrous conditions to avoid side reactions .
- Purification : Use silica gel chromatography (ethyl acetate/hexane, 3:7 ratio) to resolve acetylated derivatives .
Q. How can β-D-glucose act as a green reductant in nanoparticle synthesis?
Methodological Answer :
- Mechanism : β-D-glucose reduces Ag⁺ to Ag⁰ under mild heating (60–80°C), with starch stabilizing nanoparticles via hydroxyl group coordination .
- Critical parameters : Optimize glucose/AgNO₃ molar ratio (2:1) and pH (8–10) to control particle size (20–50 nm, validated by TEM) .
- Reproducibility : Microfluidic reactors reduce polydispersity by controlling nucleation rates .
Q. How do researchers resolve metabolic flux discrepancies in β-D-glucose 6-phosphate utilization?
Methodological Answer :
- Isotopic tracing : ¹³C-labeled glucose with metabolic flux analysis (MFA) quantifies partitioning into glycolysis vs. pentose phosphate pathways .
- Compartmentalization : Subcellular fractionation (e.g., cytosolic vs. mitochondrial G6P pools) clarifies pathway contributions .
- Enzyme profiling : Assay glucose-6-phosphate dehydrogenase (G6PDH) activity to identify regulatory nodes .
Q. Why do reported enzymatic oxidation rates of β-D-glucose vary across studies?
Methodological Answer :
- Cofactor dependency : Glucose oxidase (O₂-dependent, = 0.2 mM) vs. dehydrogenases (NAD⁺-dependent, = 5 mM) yield divergent kinetics .
- Source variability : Fungal (Aspergillus) glucose oxidase has higher thermostability than bacterial (Penicillium) isoforms .
- pH effects : Optimal activity for glucose oxidase occurs at pH 5.5–7.0; deviations alter reaction rates .
Comparison with Similar Compounds
Comparative Analysis with Structurally Similar Compounds
Alpha-D-Glucose vs. Beta-D-Glucose
Functional Implications :
- Hexokinase Specificity : C. acetobutylicum models use this compound in hexokinase reactions (ATP + this compound → ADP + this compound 6-phosphate), whereas C. thermocellum and S. cerevisiae models utilize alpha-D-glucose . This divergence reflects species-specific metabolic adaptations.
- Glycosylation : this compound’s axial C1-OH orientation reduces steric hindrance, favoring interactions with enzymes like glycogen phosphorylase .
This compound 6-Phosphate vs. Alpha-D-Glucose 6-Phosphate
Research Findings :
- In Clostridium thermocellum, this compound 6-phosphate is isomerized to alpha-D-glucose 6-phosphate via phosphoglucomutase-1, enabling glycogen synthesis .
- KEGG pathway annotations highlight species-specific preferences: L. lactis uses R00299 (D-glucose phosphorylation), while other models favor beta-specific reactions (R01600) .
This compound Derivatives and Analogues
This compound Pentaacetate :
- Properties : Soluble in organic solvents; antibacterial and antiviral activity .
- Applications : Chiral building block for nucleoside analogues (e.g., acyclovir) .
N-Acetyl-N'-beta-D-Glucopyranosyl Urea:
- Function : Inhibits glycogen phosphorylase (Ki = 2.0 µM), a therapeutic target for type 2 diabetes .
Beta-D-Glucopyranosyl Fluoride:
- Role: Non-reactive in phosphorylase activity, contrasting with alpha-D-glucopyranosyl fluoride, which mimics phosphate donors .
Metabolic and Functional Divergence in Pathways
Glycolysis and Hexokinase Variability
- This compound Utilization : In C. acetobutylicum, hexokinase (EC 2.7.1.2) specifically phosphorylates this compound, producing this compound 6-phosphate. This contrasts with models using alpha-D-glucose, underscoring evolutionary divergence in cofactor specificity .
- Pathway Alignment Tools : Tools like MPBR map this compound reactions (e.g., R01602, R01600) to alpha-D-glucose pathways (R01786, R02739), revealing conserved functionality despite structural differences .
Microbiota and this compound Metabolism
- Cecal Metabolism: this compound levels in poultry cecum correlate with Ruminococcaceae abundance, linking microbial carbohydrate degradation to host energy regulation .
- AMPK Regulation : Downregulation of AICAR (an AMPK activator) reduces this compound levels, impairing hypoglycemic responses .
Data Tables
Table 1: Key Reactions Involving this compound
Properties
IUPAC Name |
6-(hydroxymethyl)oxane-2,3,4,5-tetrol | |
---|---|---|
Details | Computed by Lexichem TK 2.7.0 (PubChem release 2021.05.07) | |
Source | PubChem | |
URL | https://pubchem.ncbi.nlm.nih.gov | |
Description | Data deposited in or computed by PubChem | |
InChI |
InChI=1S/C6H12O6/c7-1-2-3(8)4(9)5(10)6(11)12-2/h2-11H,1H2 | |
Details | Computed by InChI 1.0.6 (PubChem release 2021.05.07) | |
Source | PubChem | |
URL | https://pubchem.ncbi.nlm.nih.gov | |
Description | Data deposited in or computed by PubChem | |
InChI Key |
WQZGKKKJIJFFOK-UHFFFAOYSA-N | |
Details | Computed by InChI 1.0.6 (PubChem release 2021.05.07) | |
Source | PubChem | |
URL | https://pubchem.ncbi.nlm.nih.gov | |
Description | Data deposited in or computed by PubChem | |
Canonical SMILES |
C(C1C(C(C(C(O1)O)O)O)O)O | |
Details | Computed by OEChem 2.3.0 (PubChem release 2021.05.07) | |
Source | PubChem | |
URL | https://pubchem.ncbi.nlm.nih.gov | |
Description | Data deposited in or computed by PubChem | |
Molecular Formula |
C6H12O6 | |
Details | Computed by PubChem 2.1 (PubChem release 2021.05.07) | |
Source | PubChem | |
URL | https://pubchem.ncbi.nlm.nih.gov | |
Description | Data deposited in or computed by PubChem | |
DSSTOX Substance ID |
DTXSID80858960 | |
Record name | Hexopyranose | |
Source | EPA DSSTox | |
URL | https://comptox.epa.gov/dashboard/DTXSID80858960 | |
Description | DSSTox provides a high quality public chemistry resource for supporting improved predictive toxicology. | |
Molecular Weight |
180.16 g/mol | |
Details | Computed by PubChem 2.1 (PubChem release 2021.05.07) | |
Source | PubChem | |
URL | https://pubchem.ncbi.nlm.nih.gov | |
Description | Data deposited in or computed by PubChem | |
CAS No. |
42752-07-8, 579-36-2, 2280-44-6, 39392-65-9, 10257-28-0 | |
Record name | Hexopyranose | |
Source | CAS Common Chemistry | |
URL | https://commonchemistry.cas.org/detail?cas_rn=42752-07-8 | |
Description | CAS Common Chemistry is an open community resource for accessing chemical information. Nearly 500,000 chemical substances from CAS REGISTRY cover areas of community interest, including common and frequently regulated chemicals, and those relevant to high school and undergraduate chemistry classes. This chemical information, curated by our expert scientists, is provided in alignment with our mission as a division of the American Chemical Society. | |
Explanation | The data from CAS Common Chemistry is provided under a CC-BY-NC 4.0 license, unless otherwise stated. | |
Record name | D-Allopyranose | |
Source | DTP/NCI | |
URL | https://dtp.cancer.gov/dtpstandard/servlet/dwindex?searchtype=NSC&outputformat=html&searchlist=144657 | |
Description | The NCI Development Therapeutics Program (DTP) provides services and resources to the academic and private-sector research communities worldwide to facilitate the discovery and development of new cancer therapeutic agents. | |
Explanation | Unless otherwise indicated, all text within NCI products is free of copyright and may be reused without our permission. Credit the National Cancer Institute as the source. | |
Record name | NSC287045 | |
Source | DTP/NCI | |
URL | https://dtp.cancer.gov/dtpstandard/servlet/dwindex?searchtype=NSC&outputformat=html&searchlist=287045 | |
Description | The NCI Development Therapeutics Program (DTP) provides services and resources to the academic and private-sector research communities worldwide to facilitate the discovery and development of new cancer therapeutic agents. | |
Explanation | Unless otherwise indicated, all text within NCI products is free of copyright and may be reused without our permission. Credit the National Cancer Institute as the source. | |
Record name | NSC274237 | |
Source | DTP/NCI | |
URL | https://dtp.cancer.gov/dtpstandard/servlet/dwindex?searchtype=NSC&outputformat=html&searchlist=274237 | |
Description | The NCI Development Therapeutics Program (DTP) provides services and resources to the academic and private-sector research communities worldwide to facilitate the discovery and development of new cancer therapeutic agents. | |
Explanation | Unless otherwise indicated, all text within NCI products is free of copyright and may be reused without our permission. Credit the National Cancer Institute as the source. | |
Record name | D-galactose | |
Source | DTP/NCI | |
URL | https://dtp.cancer.gov/dtpstandard/servlet/dwindex?searchtype=NSC&outputformat=html&searchlist=8102 | |
Description | The NCI Development Therapeutics Program (DTP) provides services and resources to the academic and private-sector research communities worldwide to facilitate the discovery and development of new cancer therapeutic agents. | |
Explanation | Unless otherwise indicated, all text within NCI products is free of copyright and may be reused without our permission. Credit the National Cancer Institute as the source. | |
Record name | Hexopyranose | |
Source | EPA DSSTox | |
URL | https://comptox.epa.gov/dashboard/DTXSID80858960 | |
Description | DSSTox provides a high quality public chemistry resource for supporting improved predictive toxicology. | |
Synthesis routes and methods I
Procedure details
Synthesis routes and methods II
Procedure details
Retrosynthesis Analysis
AI-Powered Synthesis Planning: Our tool employs the Template_relevance Pistachio, Template_relevance Bkms_metabolic, Template_relevance Pistachio_ringbreaker, Template_relevance Reaxys, Template_relevance Reaxys_biocatalysis model, leveraging a vast database of chemical reactions to predict feasible synthetic routes.
One-Step Synthesis Focus: Specifically designed for one-step synthesis, it provides concise and direct routes for your target compounds, streamlining the synthesis process.
Accurate Predictions: Utilizing the extensive PISTACHIO, BKMS_METABOLIC, PISTACHIO_RINGBREAKER, REAXYS, REAXYS_BIOCATALYSIS database, our tool offers high-accuracy predictions, reflecting the latest in chemical research and data.
Strategy Settings
Precursor scoring | Relevance Heuristic |
---|---|
Min. plausibility | 0.01 |
Model | Template_relevance |
Template Set | Pistachio/Bkms_metabolic/Pistachio_ringbreaker/Reaxys/Reaxys_biocatalysis |
Top-N result to add to graph | 6 |
Feasible Synthetic Routes
Disclaimer and Information on In-Vitro Research Products
Please be aware that all articles and product information presented on BenchChem are intended solely for informational purposes. The products available for purchase on BenchChem are specifically designed for in-vitro studies, which are conducted outside of living organisms. In-vitro studies, derived from the Latin term "in glass," involve experiments performed in controlled laboratory settings using cells or tissues. It is important to note that these products are not categorized as medicines or drugs, and they have not received approval from the FDA for the prevention, treatment, or cure of any medical condition, ailment, or disease. We must emphasize that any form of bodily introduction of these products into humans or animals is strictly prohibited by law. It is essential to adhere to these guidelines to ensure compliance with legal and ethical standards in research and experimentation.