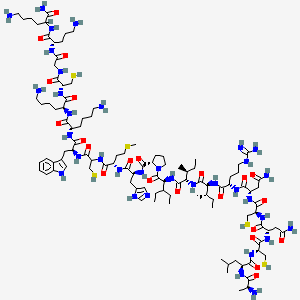
Tertia-pin (reduced)
Overview
Description
Tertia-pin (reduced) is a chemical compound that has gained significant attention in scientific research in recent years. It is a reduced form of tertia-pin, a compound that has been utilized in various applications, including as a starting material for the synthesis of other chemicals. Tertia-pin (reduced) has been found to possess unique properties that make it an attractive candidate for use in various scientific research applications.
Scientific Research Applications
I have conducted searches to find detailed scientific research applications for “Tertia-pin (reduced)” or “UNII-3A7MX9B7E8”. However, the available information is limited and does not provide a comprehensive list of unique applications. One application mentioned is that a compound named “Tertiapin” blocks certain potassium channels, which could be related to your query .
Mechanism of Action
Target of Action
Tertia-pin (reduced), also known as UNII-3A7MX9B7E8, primarily targets potassium channels . Specifically, it blocks two different types of potassium channels: inward rectifier potassium channels (Kir) and calcium-activated large conductance potassium channels (BK) . These channels play crucial roles in maintaining the resting potential, the duration of the action potential, and neuronal excitability .
Mode of Action
Tertia-pin binds specifically to different subunits of the Kir channel, namely GIRK1 (Kir 3.1), GIRK4 (Kir 3.4), and ROMK1 (Kir 1.1), inducing a dose-dependent block of the potassium current . It is thought that Tertia-pin binds to the Kir channel with its α-helix situated at the C-terminal of the peptide. This α-helix is plugged into the external end of the conduction pore, thereby blocking the channel .
Biochemical Pathways
The primary biochemical pathway affected by Tertia-pin involves the regulation of potassium ion flow across the cell membrane. By blocking Kir and BK channels, Tertia-pin disrupts the normal flow of potassium ions, which can affect a variety of cellular processes, including the maintenance of the resting potential and the regulation of action potential duration .
Pharmacokinetics
It has been observed that tertia-pin inhibits bk-type k+ channels in a use- and concentration-dependent manner . This suggests that the compound’s bioavailability and effectiveness may be influenced by factors such as dosage and frequency of administration.
Result of Action
The blockade of Kir and BK channels by Tertia-pin can have significant effects at the molecular and cellular levels. For instance, it has been found that blocking these channels can enhance the action potential firing rate . Furthermore, the blockade of these channels can alter several functional measurements, demonstrating the compound’s impact on cellular physiology .
Action Environment
The action of Tertia-pin can be influenced by various environmental factors. For example, the compound’s effectiveness in blocking potassium channels can be affected by the presence of other ions in the cellular environment
properties
IUPAC Name |
(2S)-N-[(2S)-1-[[(2S,3S)-1-[[(2S,3S)-1-[[(2S)-1-[(2S)-2-[[(2S)-1-[[(2S)-1-[[1-[[(2S)-1-[[(2S)-6-amino-1-[[(2S)-6-amino-1-[[(2R)-1-[[2-[[(2S)-5-amino-1-[(1,6-diamino-1-oxohexan-2-yl)amino]-1-oxopentan-2-yl]amino]-2-oxoethyl]amino]-1-oxo-3-sulfanylpropan-2-yl]amino]-1-oxohexan-2-yl]amino]-1-oxohexan-2-yl]amino]-3-(1H-indol-3-yl)-1-oxopropan-2-yl]amino]-1-oxo-3-sulfanylpropan-2-yl]amino]-4-methylsulfanyl-1-oxobutan-2-yl]amino]-3-(1H-imidazol-5-yl)-1-oxopropan-2-yl]carbamoyl]pyrrolidin-1-yl]-3-ethyl-1-oxopentan-2-yl]amino]-3-methyl-1-oxopentan-2-yl]amino]-3-methyl-1-oxopentan-2-yl]amino]-5-carbamimidamido-1-oxopentan-2-yl]-2-[[(2R)-2-[[(2S)-4-amino-2-[[(2R)-2-[[(2S)-2-[[(2S)-2-aminopropanoyl]amino]-4-methylpentanoyl]amino]-3-sulfanylpropanoyl]amino]-4-oxobutanoyl]amino]-3-sulfanylpropanoyl]amino]butanediamide | |
---|---|---|
Source | PubChem | |
URL | https://pubchem.ncbi.nlm.nih.gov | |
Description | Data deposited in or computed by PubChem | |
InChI |
InChI=1S/C106H180N34O23S5/c1-11-56(7)83(137-93(151)68(32-24-39-118-106(115)116)126-97(155)73(45-80(112)141)130-101(159)78(53-167)136-98(156)74(46-81(113)142)131-100(158)77(52-166)135-94(152)70(42-55(5)6)128-87(145)58(9)111)103(161)138-84(57(8)12-2)104(162)139-85(59(13-3)14-4)105(163)140-40-25-33-79(140)102(160)132-72(44-61-48-117-54-121-61)96(154)127-69(34-41-168-10)92(150)134-76(51-165)99(157)129-71(43-60-47-119-63-27-16-15-26-62(60)63)95(153)125-66(29-18-21-36-108)90(148)124-67(30-19-22-37-109)91(149)133-75(50-164)88(146)120-49-82(143)122-65(31-23-38-110)89(147)123-64(86(114)144)28-17-20-35-107/h15-16,26-27,47-48,54-59,64-79,83-85,119,164-167H,11-14,17-25,28-46,49-53,107-111H2,1-10H3,(H2,112,141)(H2,113,142)(H2,114,144)(H,117,121)(H,120,146)(H,122,143)(H,123,147)(H,124,148)(H,125,153)(H,126,155)(H,127,154)(H,128,145)(H,129,157)(H,130,159)(H,131,158)(H,132,160)(H,133,149)(H,134,150)(H,135,152)(H,136,156)(H,137,151)(H,138,161)(H,139,162)(H4,115,116,118)/t56-,57-,58-,64?,65-,66-,67-,68-,69-,70-,71-,72-,73-,74-,75-,76?,77-,78-,79-,83-,84-,85-/m0/s1 | |
Source | PubChem | |
URL | https://pubchem.ncbi.nlm.nih.gov | |
Description | Data deposited in or computed by PubChem | |
InChI Key |
QWKNGDWCZWKGME-NPHAJLTCSA-N | |
Source | PubChem | |
URL | https://pubchem.ncbi.nlm.nih.gov | |
Description | Data deposited in or computed by PubChem | |
Canonical SMILES |
CCC(C)C(C(=O)NC(C(C)CC)C(=O)NC(C(CC)CC)C(=O)N1CCCC1C(=O)NC(CC2=CN=CN2)C(=O)NC(CCSC)C(=O)NC(CS)C(=O)NC(CC3=CNC4=CC=CC=C43)C(=O)NC(CCCCN)C(=O)NC(CCCCN)C(=O)NC(CS)C(=O)NCC(=O)NC(CCCN)C(=O)NC(CCCCN)C(=O)N)NC(=O)C(CCCNC(=N)N)NC(=O)C(CC(=O)N)NC(=O)C(CS)NC(=O)C(CC(=O)N)NC(=O)C(CS)NC(=O)C(CC(C)C)NC(=O)C(C)N | |
Source | PubChem | |
URL | https://pubchem.ncbi.nlm.nih.gov | |
Description | Data deposited in or computed by PubChem | |
Isomeric SMILES |
CC[C@H](C)[C@@H](C(=O)N[C@@H]([C@@H](C)CC)C(=O)N[C@@H](C(CC)CC)C(=O)N1CCC[C@H]1C(=O)N[C@@H](CC2=CN=CN2)C(=O)N[C@@H](CCSC)C(=O)NC(CS)C(=O)N[C@@H](CC3=CNC4=CC=CC=C43)C(=O)N[C@@H](CCCCN)C(=O)N[C@@H](CCCCN)C(=O)N[C@@H](CS)C(=O)NCC(=O)N[C@@H](CCCN)C(=O)NC(CCCCN)C(=O)N)NC(=O)[C@H](CCCNC(=N)N)NC(=O)[C@H](CC(=O)N)NC(=O)[C@H](CS)NC(=O)[C@H](CC(=O)N)NC(=O)[C@H](CS)NC(=O)[C@H](CC(C)C)NC(=O)[C@H](C)N | |
Source | PubChem | |
URL | https://pubchem.ncbi.nlm.nih.gov | |
Description | Data deposited in or computed by PubChem | |
Molecular Formula |
C106H180N34O23S5 | |
Source | PubChem | |
URL | https://pubchem.ncbi.nlm.nih.gov | |
Description | Data deposited in or computed by PubChem | |
DSSTOX Substance ID |
DTXSID10897241 | |
Record name | Tertiapin | |
Source | EPA DSSTox | |
URL | https://comptox.epa.gov/dashboard/DTXSID10897241 | |
Description | DSSTox provides a high quality public chemistry resource for supporting improved predictive toxicology. | |
Molecular Weight |
2459.1 g/mol | |
Source | PubChem | |
URL | https://pubchem.ncbi.nlm.nih.gov | |
Description | Data deposited in or computed by PubChem | |
Product Name |
Tertia-pin (reduced) | |
CAS RN |
58694-52-3 | |
Record name | Tertiapin | |
Source | ChemIDplus | |
URL | https://pubchem.ncbi.nlm.nih.gov/substance/?source=chemidplus&sourceid=0058694523 | |
Description | ChemIDplus is a free, web search system that provides access to the structure and nomenclature authority files used for the identification of chemical substances cited in National Library of Medicine (NLM) databases, including the TOXNET system. | |
Record name | Tertiapin | |
Source | EPA DSSTox | |
URL | https://comptox.epa.gov/dashboard/DTXSID10897241 | |
Description | DSSTox provides a high quality public chemistry resource for supporting improved predictive toxicology. | |
Disclaimer and Information on In-Vitro Research Products
Please be aware that all articles and product information presented on BenchChem are intended solely for informational purposes. The products available for purchase on BenchChem are specifically designed for in-vitro studies, which are conducted outside of living organisms. In-vitro studies, derived from the Latin term "in glass," involve experiments performed in controlled laboratory settings using cells or tissues. It is important to note that these products are not categorized as medicines or drugs, and they have not received approval from the FDA for the prevention, treatment, or cure of any medical condition, ailment, or disease. We must emphasize that any form of bodily introduction of these products into humans or animals is strictly prohibited by law. It is essential to adhere to these guidelines to ensure compliance with legal and ethical standards in research and experimentation.