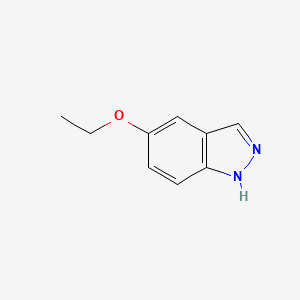
5-Ethoxy-1H-indazole
Overview
Description
5-Ethoxy-1H-indazole is a heterocyclic aromatic compound featuring a bicyclic indazole core (a fused five- and six-membered ring system) substituted with an ethoxy (-OCH₂CH₃) group at the 5-position. Notably, this compound has been listed as a discontinued product by CymitQuimica, suggesting challenges in synthesis, stability, or commercial demand .
Preparation Methods
General Synthetic Approaches to 1H-Indazole Derivatives
The synthesis of 1H-indazole derivatives typically involves cyclization strategies starting from appropriately substituted precursors such as hydrazones, aminohydrazones, or haloaryl hydrazones. Several key methodologies have been reported in the literature for synthesizing 1H-indazoles under mild, metal-free, or catalyzed conditions, which can be adapted for 5-ethoxy substitution.
Metal-Free and Mild Condition Methods
A notable method involves reacting aromatic carbonyl compounds with nitrogen sources under metal-free, mild conditions at ambient temperature, which tolerates various functional groups including alkoxy substituents like ethoxy. This approach avoids harsh acids or bases, making it suitable for sensitive groups.
Palladium-Catalyzed C-H Amination
Intramolecular ligand-free palladium-catalyzed C-H amination of aminohydrazones is an effective route to 1H-indazoles. This method allows the synthesis of substituted indazoles by preparing aminohydrazones from trifluoromethanesulfonic anhydride-activated tertiary amides, followed by cyclization.
Iodine-Promoted Cyclization
Treatment of diaryl or tert-butyl aryl ketone hydrazones with iodine in the presence of potassium iodide and sodium acetate yields 1H-indazoles via direct aryl C-H amination. This method is compatible with various substituents, including alkoxy groups.
Copper-Catalyzed Cyclization of o-Haloaryl N-Sulfonylhydrazones
Copper-mediated cyclization of o-haloaryl N-sulfonylhydrazones under mild conditions provides 1H-indazoles with good functional group tolerance. This method proceeds at relatively low temperatures with low catalyst loading and can accommodate ethoxy substituents on the aromatic ring.
Specific Preparation Routes for 5-Ethoxy-1H-indazole
While direct literature on the exact synthesis of this compound is limited, the general strategies for 1H-indazole synthesis can be adapted with ethoxy-substituted starting materials.
Starting Materials
- 5-Ethoxy-substituted aromatic carbonyl compounds : These serve as precursors for hydrazone formation.
- Hydrazine or substituted hydrazides : Used to form hydrazones or aminohydrazones.
Synthetic Route Example
Step | Reaction Type | Reagents/Conditions | Notes |
---|---|---|---|
1 | Preparation of 5-ethoxybenzaldehyde or 5-ethoxyacetophenone | Commercial or synthesized via ethoxylation of hydroxybenzaldehyde/acetophenone | Provides the ethoxy group at the 5-position |
2 | Formation of hydrazone | Reaction with hydrazine hydrate or substituted hydrazides in ethanol or other solvents | Forms hydrazone intermediate |
3 | Cyclization to 1H-indazole | Iodine/KI/NaOAc or Cu(OAc)2 catalysis under mild heating | Promotes intramolecular C-H amination and ring closure |
4 | Purification | Chromatography or recrystallization | Yields pure this compound |
This sequence is supported by the general methods described for 1H-indazole synthesis.
Optimization and Industrial Considerations
Industrial synthesis focuses on scalability, cost-effectiveness, and environmental impact. The metal-free methods or copper-catalyzed cyclizations are favored due to mild conditions and fewer toxic reagents. Solvent choice and catalyst loading are optimized to maximize yield and minimize waste.
Summary Table of Preparation Methods for this compound
Research Findings and Functional Group Compatibility
- The ethoxy group at the 5-position is well tolerated by all described synthetic methods.
- Metal-free methods avoid harsh conditions that could cleave or alter the ethoxy substituent.
- Copper and palladium catalysis provide efficient cyclization with good yields and functional group tolerance.
- The use of hydrazone intermediates is a common and versatile strategy for introducing the indazole ring system.
Chemical Reactions Analysis
Types of Reactions: 5-Ethoxy-1H-indazole can undergo various chemical reactions, including:
Oxidation: The compound can be oxidized to form corresponding oxides.
Reduction: Reduction reactions can yield different reduced forms of the compound.
Substitution: Electrophilic and nucleophilic substitution reactions can introduce various functional groups onto the indazole ring.
Common Reagents and Conditions:
Oxidation: Common oxidizing agents include potassium permanganate and chromium trioxide.
Reduction: Reducing agents such as sodium borohydride and lithium aluminum hydride are often used.
Substitution: Reagents like halogens, alkylating agents, and acylating agents are employed under controlled conditions.
Major Products: The major products formed from these reactions depend on the specific reagents and conditions used. For example, oxidation may yield indazole oxides, while substitution reactions can introduce alkyl, aryl, or acyl groups onto the indazole ring.
Scientific Research Applications
5-Ethoxy-1H-indazole has a wide range of applications in scientific research:
Chemistry: It is used as a building block for the synthesis of more complex molecules.
Biology: The compound is studied for its potential biological activities, including antimicrobial and anticancer properties.
Medicine: Research is ongoing to explore its potential as a therapeutic agent for various diseases.
Industry: It is used in the development of new materials and as a precursor for the synthesis of other valuable compounds.
Mechanism of Action
The mechanism of action of 5-Ethoxy-1H-indazole involves its interaction with specific molecular targets. The compound can bind to enzymes, receptors, or other proteins, modulating their activity. This interaction can lead to various biological effects, such as inhibition of enzyme activity or alteration of signal transduction pathways.
Comparison with Similar Compounds
Structural Features
The following table compares key structural attributes of 5-Ethoxy-1H-indazole with its analogs:
Compound Name | Substituents (Positions) | Molecular Formula | Molecular Weight (g/mol) |
---|---|---|---|
This compound | Ethoxy (5) | C₉H₁₀N₂O | 162.20 (calculated) |
5-Iodo-6-methoxy-1H-indazole | Iodo (5), Methoxy (6) | C₈H₇IN₂O | 290.06 |
5-Chloro-6-methoxy-1H-indazole | Chloro (5), Methoxy (6) | C₈H₇ClN₂O | 182.61 |
5-Iodo-1H-indazole | Iodo (5) | C₇H₅IN₂ | 244.03 |
Ethyl 5-methoxy-1H-indazole-3-carboxylate | Methoxy (5), Ethoxycarbonyl (3) | C₁₁H₁₂N₂O₃ | 220.23 |
4-Chloro-5-methoxy-1H-indazole | Chloro (4), Methoxy (5) | C₈H₇ClN₂O | 182.61 |
Key Observations :
- Substituent Position: The position of substituents significantly impacts electronic and steric properties.
- Halogen vs. Alkoxy Groups : Halogens (e.g., iodine, chlorine) introduce electron-withdrawing effects and increase molecular weight, while alkoxy groups (methoxy, ethoxy) are electron-donating and influence lipophilicity .
Physicochemical Properties
Compound Name | Melting Point (°C) | Boiling Point (°C) | Density (g/cm³) |
---|---|---|---|
5-Iodo-1H-indazole | 156 | 358.2 (predicted) | 2.082 (predicted) |
5-Chloro-6-methoxy-1H-indazole | Not reported | Not reported | Not reported |
This compound | Not reported | Not reported | Not reported |
Key Observations :
- Halogen Impact: The iodine substituent in 5-Iodo-1H-indazole increases molecular weight (244.03 g/mol) and density (2.082 g/cm³) compared to non-halogenated analogs .
- Ethoxy vs.
Biological Activity
5-Ethoxy-1H-indazole is a heterocyclic compound that has garnered attention for its potential biological activities, including antimicrobial and anticancer properties. This article explores its biological activity, mechanisms of action, and relevant research findings.
Overview of this compound
This compound belongs to the indazole family, characterized by a bicyclic structure comprising a pyrazole ring fused to a benzene ring. Its unique chemical structure allows it to interact with various biological targets, leading to diverse pharmacological effects.
Target Interactions
This compound interacts with several biomolecules, influencing various cellular processes. Notably, it has been shown to inhibit cyclooxygenase-2 (COX-2), an enzyme involved in the inflammatory response, which may contribute to its anti-inflammatory and anticancer effects.
Biochemical Pathways
The compound affects multiple biochemical pathways, including:
- Cell Growth and Proliferation : It alters cell cycle regulation and induces apoptosis in cancer cells.
- Gene Expression : Modulates the expression of genes involved in cell survival and apoptosis.
Antimicrobial Properties
Research indicates that this compound exhibits significant antimicrobial activity. A study evaluating various indazole derivatives demonstrated that some derivatives possess potent anticandidal activity against Candida albicans and C. glabrata, with effective concentrations lower than those of traditional antifungal agents .
Table 1: Antimicrobial Activity of Indazole Derivatives
Compound | Target Organism | Minimum Inhibitory Concentration (MIC) |
---|---|---|
10g | C. albicans | 100 µM |
3j | C. glabrata (miconazole-resistant) | 1 mM |
10a | C. albicans | 100 µM |
Anticancer Properties
This compound has shown promising anticancer activity across different cancer cell lines. For instance, a derivative exhibited an IC50 value of 5.15 µM against the K562 leukemia cell line, indicating potent inhibitory effects . The mechanism involves apoptosis induction and modulation of key signaling pathways, such as the p53/MDM2 pathway and inhibition of Bcl2 family members .
Table 2: Antitumor Activity of this compound Derivatives
Compound | Cell Line | IC50 (µM) | Mechanism of Action |
---|---|---|---|
6o | K562 | 5.15 | Apoptosis induction |
HEK-293 | 33.2 | Selective toxicity |
Case Studies
Recent studies have focused on the synthesis and evaluation of various indazole derivatives, including this compound. For example, a study reported that specific substitutions at the indazole scaffold significantly enhanced anticandidal and anticancer activities. These findings suggest that structural modifications can lead to compounds with improved efficacy against resistant strains and cancer cells .
Q & A
Basic Research Questions
Q. How can the synthesis of 5-Ethoxy-1H-indazole be optimized for reproducibility in academic laboratories?
- Methodological Answer : The synthesis typically involves nucleophilic substitution or coupling reactions. For example, ethoxy groups can be introduced via alkylation of hydroxyl precursors under basic conditions. Purification via column chromatography (using gradients of ethyl acetate/hexane) and characterization via -NMR (e.g., δ 1.45 ppm for ethoxy CH) and LC-MS are critical. Ensure reaction parameters (temperature, solvent polarity) are tightly controlled to minimize side products. Structural validation via X-ray crystallography (using SHELXT/SHELXL ) is recommended for confirmation.
Q. What are the best practices for characterizing the crystal structure of this compound?
- Methodological Answer : Single-crystal X-ray diffraction (SC-XRD) is the gold standard. Use SHELXL for refinement, ensuring accurate treatment of thermal parameters and hydrogen bonding. For example, the ethoxy group’s torsion angles should align with density-functional theory (DFT) predictions (e.g., B3LYP functional ). Validate against powder XRD to confirm phase purity.
Q. How should researchers handle safety protocols for this compound in laboratory settings?
- Methodological Answer : Follow guidelines from safety data sheets (SDS) for structurally similar indazoles (e.g., 2-Methoxy-5-nitro-1H-benzimidazole ). Use PPE (gloves, goggles), work in a fume hood, and store in inert atmospheres. For spills, neutralize with sodium bicarbonate and adsorb with vermiculite.
Advanced Research Questions
Q. How can computational methods resolve contradictions in experimental data for this compound’s electronic properties?
- Methodological Answer : Discrepancies in dipole moments or HOMO-LUMO gaps may arise from solvent effects or basis-set limitations. Use hybrid DFT (e.g., B3LYP with exact-exchange corrections ) and compare with experimental UV-Vis spectra. For example, if theoretical λ deviates >10 nm, re-evaluate solvation models (e.g., COSMO-RS) or include dispersion corrections.
Q. What role does chirality play in the bioactivity of this compound derivatives?
- Methodological Answer : Enantiomers can exhibit divergent binding affinities. For FGFR1 inhibition (as in related indazoles ), perform molecular dynamics (MD) simulations to analyze P-loop conformational changes. Use free-energy perturbation (FEP) to quantify ΔΔG between enantiomers. Experimentally, chiral HPLC (e.g., CHIRALPAK® IG-3 column) can separate enantiomers for IC validation.
Q. How can researchers address contradictions in enzymatic inhibition data for this compound analogs?
- Methodological Answer : Inconsistent IC values may stem from assay conditions (e.g., ATP concentration in kinase assays). Normalize data using control inhibitors (e.g., staurosporine) and apply contradiction analysis frameworks :
- Step 1 : Verify assay reproducibility (n ≥ 3).
- Step 2 : Cross-validate with orthogonal methods (e.g., SPR vs. fluorescence polarization).
- Step 3 : Use multivariate statistics to isolate confounding variables (e.g., pH, temperature).
Q. What advanced techniques elucidate the binding mechanism of this compound to protein targets?
- Methodological Answer : Combine isothermal titration calorimetry (ITC) for ΔH/ΔS determination and cryo-EM for structural insights. For example, in FGFR1, hydrogen bonds with Arg570 and π-alkyl interactions with Phe489 are critical . MD simulations (AMBER or GROMACS) can predict residence times and entropy-driven binding.
Q. How can researchers validate the purity of this compound in complex matrices?
- Methodological Answer : Use orthogonal techniques:
- HPLC-DAD : Monitor λ at 254 nm; compare retention times with standards.
- HRMS : Confirm molecular ion ([M+H]) with <2 ppm error.
- -NMR : Detect trace impurities (e.g., unreacted precursors) at δ 110–160 ppm.
Properties
IUPAC Name |
5-ethoxy-1H-indazole | |
---|---|---|
Source | PubChem | |
URL | https://pubchem.ncbi.nlm.nih.gov | |
Description | Data deposited in or computed by PubChem | |
InChI |
InChI=1S/C9H10N2O/c1-2-12-8-3-4-9-7(5-8)6-10-11-9/h3-6H,2H2,1H3,(H,10,11) | |
Source | PubChem | |
URL | https://pubchem.ncbi.nlm.nih.gov | |
Description | Data deposited in or computed by PubChem | |
InChI Key |
BAAOULLTESKBQV-UHFFFAOYSA-N | |
Source | PubChem | |
URL | https://pubchem.ncbi.nlm.nih.gov | |
Description | Data deposited in or computed by PubChem | |
Canonical SMILES |
CCOC1=CC2=C(C=C1)NN=C2 | |
Source | PubChem | |
URL | https://pubchem.ncbi.nlm.nih.gov | |
Description | Data deposited in or computed by PubChem | |
Molecular Formula |
C9H10N2O | |
Source | PubChem | |
URL | https://pubchem.ncbi.nlm.nih.gov | |
Description | Data deposited in or computed by PubChem | |
DSSTOX Substance ID |
DTXSID70622091 | |
Record name | 5-Ethoxy-1H-indazole | |
Source | EPA DSSTox | |
URL | https://comptox.epa.gov/dashboard/DTXSID70622091 | |
Description | DSSTox provides a high quality public chemistry resource for supporting improved predictive toxicology. | |
Molecular Weight |
162.19 g/mol | |
Source | PubChem | |
URL | https://pubchem.ncbi.nlm.nih.gov | |
Description | Data deposited in or computed by PubChem | |
CAS No. |
518990-35-7 | |
Record name | 5-Ethoxy-1H-indazole | |
Source | EPA DSSTox | |
URL | https://comptox.epa.gov/dashboard/DTXSID70622091 | |
Description | DSSTox provides a high quality public chemistry resource for supporting improved predictive toxicology. | |
Synthesis routes and methods I
Procedure details
Synthesis routes and methods II
Procedure details
Retrosynthesis Analysis
AI-Powered Synthesis Planning: Our tool employs the Template_relevance Pistachio, Template_relevance Bkms_metabolic, Template_relevance Pistachio_ringbreaker, Template_relevance Reaxys, Template_relevance Reaxys_biocatalysis model, leveraging a vast database of chemical reactions to predict feasible synthetic routes.
One-Step Synthesis Focus: Specifically designed for one-step synthesis, it provides concise and direct routes for your target compounds, streamlining the synthesis process.
Accurate Predictions: Utilizing the extensive PISTACHIO, BKMS_METABOLIC, PISTACHIO_RINGBREAKER, REAXYS, REAXYS_BIOCATALYSIS database, our tool offers high-accuracy predictions, reflecting the latest in chemical research and data.
Strategy Settings
Precursor scoring | Relevance Heuristic |
---|---|
Min. plausibility | 0.01 |
Model | Template_relevance |
Template Set | Pistachio/Bkms_metabolic/Pistachio_ringbreaker/Reaxys/Reaxys_biocatalysis |
Top-N result to add to graph | 6 |
Feasible Synthetic Routes
Disclaimer and Information on In-Vitro Research Products
Please be aware that all articles and product information presented on BenchChem are intended solely for informational purposes. The products available for purchase on BenchChem are specifically designed for in-vitro studies, which are conducted outside of living organisms. In-vitro studies, derived from the Latin term "in glass," involve experiments performed in controlled laboratory settings using cells or tissues. It is important to note that these products are not categorized as medicines or drugs, and they have not received approval from the FDA for the prevention, treatment, or cure of any medical condition, ailment, or disease. We must emphasize that any form of bodily introduction of these products into humans or animals is strictly prohibited by law. It is essential to adhere to these guidelines to ensure compliance with legal and ethical standards in research and experimentation.