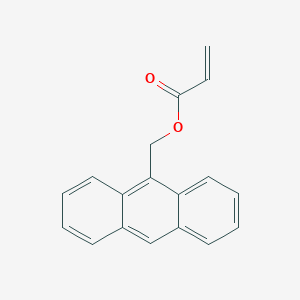
9-Anthracenylmethyl acrylate
Overview
Description
9-Anthracenylmethyl acrylate is an organic compound with the chemical formula C₁₈H₁₄O₂. It is a yellow to orange solid with a distinctive fragrance . This compound is known for its fluorescent properties and is used in various scientific and industrial applications .
Mechanism of Action
Target of Action
9-Anthracenylmethyl acrylate is a fluorescent monomer . It is used in the development of photonic and optical materials . .
Mode of Action
The compound’s mode of action is primarily through its fluorescent properties . It can absorb light at a specific wavelength and then re-emit light at a longer wavelength. This property is utilized in the development of photonic and optical materials .
Result of Action
The primary result of the action of this compound is the emission of light . This is due to its fluorescent properties, which allow it to absorb light at one wavelength and re-emit it at a longer wavelength .
Action Environment
The action of this compound is influenced by environmental factors such as light and temperature. For instance, its fluorescent properties are triggered by light . Additionally, its stability may be affected by heat, as suggested by its melting point of 80-85 °C .
Biochemical Analysis
Biochemical Properties
It is known to participate in light-triggered dimerization and heat depolymerization reactions
Cellular Effects
It has been used in the development of light- and heat-triggered reversible luminescent materials . Its influence on cell function, signaling pathways, gene expression, and cellular metabolism is yet to be explored.
Molecular Mechanism
The molecular mechanism of 9-Anthracenylmethyl acrylate involves light-triggered dimerization and heat depolymerization . It is suggested that this compound efficiently dimerizes through the photodimerization of the anthryl groups in organic solvents . The covalent bonds between pendant anthryl groups are cleaved after heating at 120 °C .
Temporal Effects in Laboratory Settings
In laboratory settings, the effects of this compound can be manipulated over time through light and heat. Upon UV light excitation, it undergoes dimerization, and upon heating at 120 °C, it undergoes depolymerization . Information on its stability, degradation, and long-term effects on cellular function is currently limited.
Preparation Methods
Synthetic Routes and Reaction Conditions: 9-Anthracenylmethyl acrylate can be synthesized through the esterification of 9-anthracenemethanol with acrylic acid. The reaction typically involves the use of a catalyst such as sulfuric acid or p-toluenesulfonic acid to facilitate the esterification process. The reaction is carried out under reflux conditions, and the product is purified through recrystallization .
Industrial Production Methods: In an industrial setting, the production of this compound may involve continuous flow reactors to optimize the reaction conditions and increase yield. The use of advanced purification techniques such as column chromatography and distillation ensures the high purity of the final product .
Types of Reactions:
Oxidation: this compound can undergo oxidation reactions to form anthraquinone derivatives.
Reduction: Reduction of this compound can lead to the formation of 9-anthracenemethanol.
Common Reagents and Conditions:
Oxidation: Potassium permanganate in an acidic medium.
Reduction: Lithium aluminum hydride in anhydrous ether.
Substitution: Amines or thiols in the presence of a base such as triethylamine.
Major Products Formed:
Oxidation: Anthraquinone derivatives.
Reduction: 9-Anthracenemethanol.
Substitution: Various substituted anthracene derivatives.
Scientific Research Applications
9-Anthracenylmethyl acrylate is utilized in a wide range of scientific research applications:
Comparison with Similar Compounds
9-Anthracenylmethyl methacrylate: Similar in structure but with a methacrylate group instead of an acrylate group.
9-Anthracenemethanol: The alcohol derivative of 9-anthracenylmethyl acrylate.
2-Naphthyl methacrylate: Another fluorescent monomer with a naphthalene moiety instead of anthracene.
Uniqueness: this compound is unique due to its combination of fluorescent properties and reactivity. The presence of the acrylate group allows for polymerization and other chemical modifications, making it versatile for various applications in materials science and biological research .
Properties
IUPAC Name |
anthracen-9-ylmethyl prop-2-enoate | |
---|---|---|
Source | PubChem | |
URL | https://pubchem.ncbi.nlm.nih.gov | |
Description | Data deposited in or computed by PubChem | |
InChI |
InChI=1S/C18H14O2/c1-2-18(19)20-12-17-15-9-5-3-7-13(15)11-14-8-4-6-10-16(14)17/h2-11H,1,12H2 | |
Source | PubChem | |
URL | https://pubchem.ncbi.nlm.nih.gov | |
Description | Data deposited in or computed by PubChem | |
InChI Key |
IPLSGZRALKDNRS-UHFFFAOYSA-N | |
Source | PubChem | |
URL | https://pubchem.ncbi.nlm.nih.gov | |
Description | Data deposited in or computed by PubChem | |
Canonical SMILES |
C=CC(=O)OCC1=C2C=CC=CC2=CC3=CC=CC=C31 | |
Source | PubChem | |
URL | https://pubchem.ncbi.nlm.nih.gov | |
Description | Data deposited in or computed by PubChem | |
Molecular Formula |
C18H14O2 | |
Source | PubChem | |
URL | https://pubchem.ncbi.nlm.nih.gov | |
Description | Data deposited in or computed by PubChem | |
DSSTOX Substance ID |
DTXSID50398944 | |
Record name | 9-Anthracenylmethyl acrylate | |
Source | EPA DSSTox | |
URL | https://comptox.epa.gov/dashboard/DTXSID50398944 | |
Description | DSSTox provides a high quality public chemistry resource for supporting improved predictive toxicology. | |
Molecular Weight |
262.3 g/mol | |
Source | PubChem | |
URL | https://pubchem.ncbi.nlm.nih.gov | |
Description | Data deposited in or computed by PubChem | |
CAS No. |
31645-34-8 | |
Record name | 9-Anthracenylmethyl acrylate | |
Source | EPA DSSTox | |
URL | https://comptox.epa.gov/dashboard/DTXSID50398944 | |
Description | DSSTox provides a high quality public chemistry resource for supporting improved predictive toxicology. | |
Synthesis routes and methods
Procedure details
Retrosynthesis Analysis
AI-Powered Synthesis Planning: Our tool employs the Template_relevance Pistachio, Template_relevance Bkms_metabolic, Template_relevance Pistachio_ringbreaker, Template_relevance Reaxys, Template_relevance Reaxys_biocatalysis model, leveraging a vast database of chemical reactions to predict feasible synthetic routes.
One-Step Synthesis Focus: Specifically designed for one-step synthesis, it provides concise and direct routes for your target compounds, streamlining the synthesis process.
Accurate Predictions: Utilizing the extensive PISTACHIO, BKMS_METABOLIC, PISTACHIO_RINGBREAKER, REAXYS, REAXYS_BIOCATALYSIS database, our tool offers high-accuracy predictions, reflecting the latest in chemical research and data.
Strategy Settings
Precursor scoring | Relevance Heuristic |
---|---|
Min. plausibility | 0.01 |
Model | Template_relevance |
Template Set | Pistachio/Bkms_metabolic/Pistachio_ringbreaker/Reaxys/Reaxys_biocatalysis |
Top-N result to add to graph | 6 |
Feasible Synthetic Routes
Q1: How does 9-Anthracenylmethyl acrylate contribute to the formation of reversible silicone elastomers?
A1: this compound plays a crucial role in creating reversible crosslinking networks within the silicone elastomer. The anthracene moiety within the molecule undergoes [4π–4π photo-cycloadditions] when exposed to UV light (365 nm) []. This reaction forms covalent bonds with other anthracene groups present in the material, effectively crosslinking the polymer chains and solidifying the elastomer. Importantly, this crosslinking is reversible. Upon heating to 120 °C, the photodimerization process is reversed, breaking the anthracene-anthracene bonds and returning the material to a more fluid state []. This cycle of light-induced crosslinking and heat-induced de-crosslinking allows for the development of dynamic, responsive materials.
Disclaimer and Information on In-Vitro Research Products
Please be aware that all articles and product information presented on BenchChem are intended solely for informational purposes. The products available for purchase on BenchChem are specifically designed for in-vitro studies, which are conducted outside of living organisms. In-vitro studies, derived from the Latin term "in glass," involve experiments performed in controlled laboratory settings using cells or tissues. It is important to note that these products are not categorized as medicines or drugs, and they have not received approval from the FDA for the prevention, treatment, or cure of any medical condition, ailment, or disease. We must emphasize that any form of bodily introduction of these products into humans or animals is strictly prohibited by law. It is essential to adhere to these guidelines to ensure compliance with legal and ethical standards in research and experimentation.