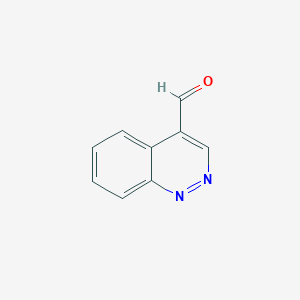
Cinnoline-4-carbaldehyde
Overview
Description
Cinnoline-4-carbaldehyde is a heterocyclic aromatic compound that belongs to the cinnoline family. Cinnolines are nitrogen-containing bicyclic compounds that have significant pharmacological and chemical importance. The structure of this compound consists of a cinnoline ring system with an aldehyde functional group at the fourth position. This compound is known for its diverse applications in medicinal chemistry, synthetic organic chemistry, and material science.
Mechanism of Action
Target of Action
Cinnoline-4-carbaldehyde is a derivative of cinnoline, a type of fused N-heterocyclic compound . These compounds are known to have a broad range of biological properties and are used as synthetic intermediates, potential drug candidates, and chemical probes . .
Mode of Action
It’s known that cinnoline derivatives exhibit a broad spectrum of pharmacological activities such as antibacterial, antifungal, antimalarial, anti-inflammatory, analgesic, anxiolytic, and antitumor activities
Biochemical Pathways
This compound, as a cinnoline derivative, is part of a larger group of compounds known as N-heterocyclic compounds. These compounds play an essential role in natural systems and are involved in various biochemical pathways . .
Pharmacokinetics
It’s known that the development of cinnoline-based molecules contributes significantly to the identification of lead compounds with optimized pharmacodynamic and pharmacokinetic properties .
Result of Action
Cinnoline derivatives are known to exhibit a broad spectrum of pharmacological activities
Action Environment
It’s known that the synthesis of cinnoline derivatives can be influenced by various factors, including the reaction environment .
Preparation Methods
Synthetic Routes and Reaction Conditions: Cinnoline-4-carbaldehyde can be synthesized through various methods. One common approach involves the diazotization of 2-aminobenzaldehyde followed by cyclization. The reaction typically requires acidic conditions and a diazotizing agent such as sodium nitrite. Another method involves the cyclization of 2-aminobenzyl alcohol derivatives under oxidative conditions.
Industrial Production Methods: In industrial settings, this compound is often produced using metal-catalyzed reactions. For example, palladium-catalyzed cross-coupling reactions can be employed to form the cinnoline ring system. These methods are advantageous due to their high yield and scalability.
Chemical Reactions Analysis
Types of Reactions: Cinnoline-4-carbaldehyde undergoes various chemical reactions, including:
Oxidation: The aldehyde group can be oxidized to form cinnoline-4-carboxylic acid.
Reduction: The aldehyde group can be reduced to form cinnoline-4-methanol.
Common Reagents and Conditions:
Oxidation: Common oxidizing agents include potassium permanganate and chromium trioxide.
Reduction: Reducing agents such as sodium borohydride and lithium aluminum hydride are typically used.
Substitution: Electrophilic reagents such as halogens and nitrating agents can be used under acidic or basic conditions.
Major Products:
Oxidation: Cinnoline-4-carboxylic acid.
Reduction: Cinnoline-4-methanol.
Substitution: Various substituted cinnoline derivatives depending on the electrophile used.
Scientific Research Applications
Cinnoline-4-carbaldehyde has a wide range of applications in scientific research:
Chemistry: It is used as a building block for the synthesis of more complex heterocyclic compounds.
Biology: Cinnoline derivatives have shown potential as antimicrobial and anticancer agents.
Medicine: Some cinnoline-based compounds are being investigated for their therapeutic properties, including anti-inflammatory and analgesic effects.
Industry: this compound is used in the development of dyes, pigments, and advanced materials.
Comparison with Similar Compounds
- Quinoline
- Isoquinoline
- Phthalazine
- Quinoxaline
- Quinazoline
Cinnoline-4-carbaldehyde stands out due to its versatile reactivity and wide range of applications in various fields of research and industry.
Properties
IUPAC Name |
cinnoline-4-carbaldehyde | |
---|---|---|
Source | PubChem | |
URL | https://pubchem.ncbi.nlm.nih.gov | |
Description | Data deposited in or computed by PubChem | |
InChI |
InChI=1S/C9H6N2O/c12-6-7-5-10-11-9-4-2-1-3-8(7)9/h1-6H | |
Source | PubChem | |
URL | https://pubchem.ncbi.nlm.nih.gov | |
Description | Data deposited in or computed by PubChem | |
InChI Key |
CORTZCFBTFGJGU-UHFFFAOYSA-N | |
Source | PubChem | |
URL | https://pubchem.ncbi.nlm.nih.gov | |
Description | Data deposited in or computed by PubChem | |
Canonical SMILES |
C1=CC=C2C(=C1)C(=CN=N2)C=O | |
Source | PubChem | |
URL | https://pubchem.ncbi.nlm.nih.gov | |
Description | Data deposited in or computed by PubChem | |
Molecular Formula |
C9H6N2O | |
Source | PubChem | |
URL | https://pubchem.ncbi.nlm.nih.gov | |
Description | Data deposited in or computed by PubChem | |
DSSTOX Substance ID |
DTXSID50595633 | |
Record name | Cinnoline-4-carbaldehyde | |
Source | EPA DSSTox | |
URL | https://comptox.epa.gov/dashboard/DTXSID50595633 | |
Description | DSSTox provides a high quality public chemistry resource for supporting improved predictive toxicology. | |
Molecular Weight |
158.16 g/mol | |
Source | PubChem | |
URL | https://pubchem.ncbi.nlm.nih.gov | |
Description | Data deposited in or computed by PubChem | |
CAS No. |
90418-57-8 | |
Record name | Cinnoline-4-carbaldehyde | |
Source | EPA DSSTox | |
URL | https://comptox.epa.gov/dashboard/DTXSID50595633 | |
Description | DSSTox provides a high quality public chemistry resource for supporting improved predictive toxicology. | |
Retrosynthesis Analysis
AI-Powered Synthesis Planning: Our tool employs the Template_relevance Pistachio, Template_relevance Bkms_metabolic, Template_relevance Pistachio_ringbreaker, Template_relevance Reaxys, Template_relevance Reaxys_biocatalysis model, leveraging a vast database of chemical reactions to predict feasible synthetic routes.
One-Step Synthesis Focus: Specifically designed for one-step synthesis, it provides concise and direct routes for your target compounds, streamlining the synthesis process.
Accurate Predictions: Utilizing the extensive PISTACHIO, BKMS_METABOLIC, PISTACHIO_RINGBREAKER, REAXYS, REAXYS_BIOCATALYSIS database, our tool offers high-accuracy predictions, reflecting the latest in chemical research and data.
Strategy Settings
Precursor scoring | Relevance Heuristic |
---|---|
Min. plausibility | 0.01 |
Model | Template_relevance |
Template Set | Pistachio/Bkms_metabolic/Pistachio_ringbreaker/Reaxys/Reaxys_biocatalysis |
Top-N result to add to graph | 6 |
Feasible Synthetic Routes
Disclaimer and Information on In-Vitro Research Products
Please be aware that all articles and product information presented on BenchChem are intended solely for informational purposes. The products available for purchase on BenchChem are specifically designed for in-vitro studies, which are conducted outside of living organisms. In-vitro studies, derived from the Latin term "in glass," involve experiments performed in controlled laboratory settings using cells or tissues. It is important to note that these products are not categorized as medicines or drugs, and they have not received approval from the FDA for the prevention, treatment, or cure of any medical condition, ailment, or disease. We must emphasize that any form of bodily introduction of these products into humans or animals is strictly prohibited by law. It is essential to adhere to these guidelines to ensure compliance with legal and ethical standards in research and experimentation.