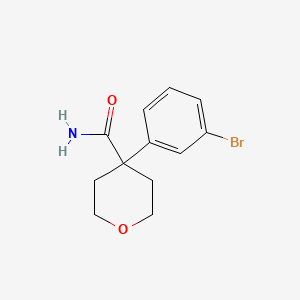
4-(3-Bromophenyl)tetrahydro-2H-pyran-4-carboxamide
Overview
Description
4-(3-Bromophenyl)tetrahydro-2H-pyran-4-carboxamide is a brominated tetrahydro-2H-pyran derivative featuring a carboxamide group at the 4-position and a 3-bromophenyl substituent. It serves as a critical intermediate in synthesizing 5-lipoxygenase (5-LO) inhibitors, such as PF-04191834, a therapeutic candidate for osteoarthritis . Key properties include:
Preparation Methods
Synthetic Routes and Reaction Conditions
The synthesis of 4-(3-Bromophenyl)tetrahydro-2H-pyran-4-carboxamide typically involves the following steps:
Formation of the Tetrahydropyran Ring: The tetrahydropyran ring can be synthesized through the acid-catalyzed cyclization of 1,5-hexanediol.
Amidation: The carboxamide group can be introduced by reacting the bromophenyl-tetrahydropyran intermediate with an appropriate amine under suitable conditions, such as using a coupling reagent like EDCI (1-ethyl-3-(3-dimethylaminopropyl)carbodiimide) in the presence of a base.
Industrial Production Methods
Industrial production of this compound may involve similar synthetic routes but on a larger scale, with optimizations for yield, purity, and cost-effectiveness. This could include the use of continuous flow reactors, automated synthesis, and advanced purification techniques.
Chemical Reactions Analysis
Types of Reactions
4-(3-Bromophenyl)tetrahydro-2H-pyran-4-carboxamide can undergo various chemical reactions, including:
Substitution Reactions: The bromine atom on the phenyl ring can be substituted with other nucleophiles, such as amines, thiols, or alkoxides, under appropriate conditions.
Oxidation and Reduction: The compound can undergo oxidation or reduction reactions, depending on the functional groups present and the reagents used.
Hydrolysis: The carboxamide group can be hydrolyzed under acidic or basic conditions to yield the corresponding carboxylic acid and amine.
Common Reagents and Conditions
Substitution Reactions: Common reagents include sodium azide, potassium thiolate, and sodium alkoxide. Conditions typically involve the use of polar aprotic solvents like dimethylformamide (DMF) or dimethyl sulfoxide (DMSO) and elevated temperatures.
Oxidation: Reagents such as potassium permanganate (KMnO4) or chromium trioxide (CrO3) can be used under acidic conditions.
Reduction: Reducing agents like lithium aluminum hydride (LiAlH4) or sodium borohydride (NaBH4) are commonly employed.
Hydrolysis: Acidic hydrolysis can be performed using hydrochloric acid (HCl) or sulfuric acid (H2SO4), while basic hydrolysis can be achieved with sodium hydroxide (NaOH) or potassium hydroxide (KOH).
Major Products Formed
Substitution: Products depend on the nucleophile used, resulting in derivatives such as azides, thiols, or ethers.
Oxidation: Oxidized products may include carboxylic acids or ketones.
Reduction: Reduced products may include alcohols or amines.
Hydrolysis: Hydrolysis yields the corresponding carboxylic acid and amine.
Scientific Research Applications
4-(3-Bromophenyl)tetrahydro-2H-pyran-4-carboxamide has several scientific research applications:
Organic Synthesis: It serves as a building block for the synthesis of more complex molecules, including pharmaceuticals and agrochemicals.
Medicinal Chemistry: The compound is investigated for its potential biological activities, such as antimicrobial, anticancer, and anti-inflammatory properties.
Material Science: It can be used in the development of novel materials with specific properties, such as polymers or liquid crystals.
Biological Studies: The compound is used in biochemical assays to study enzyme interactions, receptor binding, and cellular pathways.
Mechanism of Action
The mechanism of action of 4-(3-Bromophenyl)tetrahydro-2H-pyran-4-carboxamide depends on its specific application. In medicinal chemistry, it may interact with molecular targets such as enzymes, receptors, or ion channels, modulating their activity. The bromophenyl group can enhance binding affinity through halogen bonding, while the tetrahydropyran ring and carboxamide group contribute to the overall molecular stability and solubility.
Comparison with Similar Compounds
Comparison with Structural Analogs
Structural Modifications and Substituent Effects
The following table summarizes key analogs and their distinguishing features:
Key Observations:
Substituent Impact on Bioactivity: The 3-bromophenyl group in the target compound likely enhances hydrophobic interactions in enzyme binding, critical for 5-LO inhibition . In contrast, the imidazole-benzyloxy substituent in the analog from improves oral bioavailability and reduces toxicity, making it a clinical candidate . Cyano groups (e.g., in Compound 9e) may increase metabolic stability but reduce solubility compared to carboxamides .
The target compound’s lower molecular weight (256 g/mol) may favor better diffusion in biological systems . Aldehyde-containing analogs () are reactive and less stable than carboxamides, limiting their utility in drug development .
Pharmacokinetic (PK) and Toxicity Profiles
- The imidazole-containing analog () demonstrates superior PK properties, attributed to fluorine’s electronegativity and imidazole’s hydrogen-bonding capacity .
Biological Activity
4-(3-Bromophenyl)tetrahydro-2H-pyran-4-carboxamide is a compound belonging to the tetrahydropyran class, characterized by its unique structural features that contribute to its biological activities. This compound has garnered interest in medicinal chemistry due to its potential pharmacological applications, including anti-inflammatory, analgesic, and antimicrobial properties.
- Molecular Formula : C₁₂H₁₄BrNO₂
- Molecular Weight : 284.149 g/mol
The presence of the bromophenyl group enhances the compound's lipophilicity and reactivity, influencing its interaction with various biological targets. The tetrahydropyran scaffold is commonly found in biologically active compounds, further supporting its potential in drug development.
Biological Activities
Research indicates that compounds similar to this compound exhibit a range of biological activities:
- Anti-inflammatory Activity : Compounds in the tetrahydropyran class have shown promise in inhibiting inflammatory pathways, potentially through the modulation of leukotriene synthesis .
- Analgesic Properties : The structural characteristics suggest that this compound may interact with pain pathways, providing analgesic effects similar to other tetrahydropyran derivatives.
- Antimicrobial Effects : Preliminary studies indicate that this compound could exhibit antimicrobial properties, particularly against Gram-positive bacteria, making it a candidate for further investigation in infectious disease contexts .
Structure-Activity Relationship (SAR)
The biological activity of this compound can be influenced by various structural modifications. A comparative analysis with similar compounds reveals insights into how different substituents affect activity:
Compound Name | Structure | Unique Features |
---|---|---|
4-(4-Bromophenyl)tetrahydro-2H-pyran-4-carboxylic acid | Structure | Contains a carboxylic acid instead of an amide |
Methyl 4-(3-bromophenyl)tetrahydro-2H-pyran-4-carboxylate | Structure | Ester derivative with altered biological activity |
4-(3-Chlorophenyl)tetrahydro-2H-pyran-4-carboxamide | Structure | Chlorine substitution may affect potency |
The bromine atom's presence in the structure is significant as it enhances lipophilicity and may influence binding interactions with biological targets compared to compounds with different halogens or functional groups.
Q & A
Q. Basic: What spectroscopic methods are recommended for confirming the structural identity of 4-(3-Bromophenyl)tetrahydro-2H-pyran-4-carboxamide?
Answer:
- 1H NMR : Analyze proton environments to confirm substituent positions and stereochemistry. For example, characteristic splitting patterns (e.g., δ 7.62 ppm for aromatic protons adjacent to bromine) and integration ratios can validate the 3-bromophenyl group and tetrahydro-2H-pyran ring .
- HRMS : Use high-resolution mass spectrometry to confirm molecular formula (e.g., [M+H]+ with <3 ppm error ensures accuracy). Reported HRMS data for similar compounds show deviations as low as 2.8 ppm .
- Elemental Analysis : Compare experimental vs. calculated C, H, N percentages (e.g., Anal. calc’d/found discrepancies ≤0.3% validate purity) .
Q. Advanced: How can researchers resolve contradictions in spectral data during structural elucidation?
Answer:
- Orthogonal Techniques : Cross-validate NMR with 13C NMR, IR (e.g., carboxamide C=O stretch ~1650 cm⁻¹), and X-ray crystallography if crystalline .
- Computational Validation : Use DFT calculations to simulate NMR spectra and compare with experimental data. Tools like Gaussian or ORCA can predict chemical shifts for bromine-induced deshielding effects .
- Dynamic Exchange Analysis : For ambiguous splitting patterns, variable-temperature NMR can distinguish between conformational dynamics and impurities .
Q. Basic: What synthetic strategies are feasible for introducing substituents to the tetrahydro-2H-pyran ring?
Answer:
- Retrosynthetic Planning : Target the pyran ring via cyclization of diols or ketones. For example, 4-position substitution can be achieved using 4-amino-tetrahydro-2H-pyran-4-carbonitrile as a precursor .
- Functionalization Post-Cyclization : Bromophenyl groups are typically introduced via Suzuki-Miyaura coupling or nucleophilic aromatic substitution .
Q. Advanced: How can AI-driven synthesis planning improve route efficiency for novel derivatives?
Answer:
- Template-Based Models : Platforms like Pistachio or Reaxys databases predict feasible reactions by matching molecular fragments to known templates. For example, a bromophenyl group can be prioritized for cross-coupling reactions .
- Reaxys Ring Breaker : Identifies strategic bond cleavages to simplify retrosynthesis (e.g., opening the pyran ring to introduce substituents) .
- Feedback Loop Integration : Combine quantum chemical calculations (e.g., activation energy barriers) with experimental yields to refine route predictions .
Q. Basic: What experimental design principles optimize reaction conditions for carboxamide formation?
Answer:
- Design of Experiments (DoE) : Use factorial designs to test variables (temperature, solvent, catalyst). For example, a 2³ factorial design can optimize amidation yield by varying DMF/THF ratios, 50–100°C, and 1–5 mol% DMAP .
- Response Surface Methodology (RSM) : Model non-linear relationships between variables (e.g., solvent polarity vs. carboxamide crystallinity) .
Q. Advanced: How to address low reproducibility in multi-step syntheses?
Answer:
- Process Analytical Technology (PAT) : Implement in-situ FTIR or Raman spectroscopy to monitor intermediates (e.g., carboxamide formation in real-time) .
- Critical Parameter Identification : Use sensitivity analysis to rank factors (e.g., moisture sensitivity of bromophenyl intermediates) .
- Scale-Down Models : Mimic large-scale conditions in microreactors to preempt mixing inefficiencies .
Q. Basic: What purification methods are effective for isolating this compound?
Answer:
- Column Chromatography : Use silica gel with gradient elution (e.g., hexane/EtOAc 3:1 to 1:2) to separate bromophenyl byproducts .
- Recrystallization : Optimize solvent pairs (e.g., EtOH/H2O) based on solubility data. Purity >95% is achievable with iterative recrystallization .
Q. Advanced: How to evaluate the compound’s bioactivity against HMG-CoA reductase?
Answer:
- Enzyme Assays : Use spectrophotometric methods to measure NADPH consumption at 340 nm. Compare IC50 values with positive controls (e.g., pitavastatin) .
- Docking Simulations : AutoDock Vina or Schrödinger Suite can predict binding affinities to the reductase’s active site, guided by the bromophenyl group’s hydrophobicity .
Q. Basic: What safety protocols are critical when handling brominated intermediates?
Answer:
- Personal Protective Equipment (PPE) : Use nitrile gloves and fume hoods to avoid dermal/airborne exposure .
- Waste Management : Neutralize brominated waste with NaHCO3 before disposal .
Q. Advanced: How to design a reactor for scalable synthesis while minimizing hazards?
Answer:
Properties
IUPAC Name |
4-(3-bromophenyl)oxane-4-carboxamide | |
---|---|---|
Source | PubChem | |
URL | https://pubchem.ncbi.nlm.nih.gov | |
Description | Data deposited in or computed by PubChem | |
InChI |
InChI=1S/C12H14BrNO2/c13-10-3-1-2-9(8-10)12(11(14)15)4-6-16-7-5-12/h1-3,8H,4-7H2,(H2,14,15) | |
Source | PubChem | |
URL | https://pubchem.ncbi.nlm.nih.gov | |
Description | Data deposited in or computed by PubChem | |
InChI Key |
ZBBPOKGRGPCSHF-UHFFFAOYSA-N | |
Source | PubChem | |
URL | https://pubchem.ncbi.nlm.nih.gov | |
Description | Data deposited in or computed by PubChem | |
Canonical SMILES |
C1COCCC1(C2=CC(=CC=C2)Br)C(=O)N | |
Source | PubChem | |
URL | https://pubchem.ncbi.nlm.nih.gov | |
Description | Data deposited in or computed by PubChem | |
Molecular Formula |
C12H14BrNO2 | |
Source | PubChem | |
URL | https://pubchem.ncbi.nlm.nih.gov | |
Description | Data deposited in or computed by PubChem | |
DSSTOX Substance ID |
DTXSID40573960 | |
Record name | 4-(3-Bromophenyl)oxane-4-carboxamide | |
Source | EPA DSSTox | |
URL | https://comptox.epa.gov/dashboard/DTXSID40573960 | |
Description | DSSTox provides a high quality public chemistry resource for supporting improved predictive toxicology. | |
Molecular Weight |
284.15 g/mol | |
Source | PubChem | |
URL | https://pubchem.ncbi.nlm.nih.gov | |
Description | Data deposited in or computed by PubChem | |
CAS No. |
329025-26-5 | |
Record name | 4-(3-Bromophenyl)oxane-4-carboxamide | |
Source | EPA DSSTox | |
URL | https://comptox.epa.gov/dashboard/DTXSID40573960 | |
Description | DSSTox provides a high quality public chemistry resource for supporting improved predictive toxicology. | |
Synthesis routes and methods I
Procedure details
Synthesis routes and methods II
Procedure details
Retrosynthesis Analysis
AI-Powered Synthesis Planning: Our tool employs the Template_relevance Pistachio, Template_relevance Bkms_metabolic, Template_relevance Pistachio_ringbreaker, Template_relevance Reaxys, Template_relevance Reaxys_biocatalysis model, leveraging a vast database of chemical reactions to predict feasible synthetic routes.
One-Step Synthesis Focus: Specifically designed for one-step synthesis, it provides concise and direct routes for your target compounds, streamlining the synthesis process.
Accurate Predictions: Utilizing the extensive PISTACHIO, BKMS_METABOLIC, PISTACHIO_RINGBREAKER, REAXYS, REAXYS_BIOCATALYSIS database, our tool offers high-accuracy predictions, reflecting the latest in chemical research and data.
Strategy Settings
Precursor scoring | Relevance Heuristic |
---|---|
Min. plausibility | 0.01 |
Model | Template_relevance |
Template Set | Pistachio/Bkms_metabolic/Pistachio_ringbreaker/Reaxys/Reaxys_biocatalysis |
Top-N result to add to graph | 6 |
Feasible Synthetic Routes
Disclaimer and Information on In-Vitro Research Products
Please be aware that all articles and product information presented on BenchChem are intended solely for informational purposes. The products available for purchase on BenchChem are specifically designed for in-vitro studies, which are conducted outside of living organisms. In-vitro studies, derived from the Latin term "in glass," involve experiments performed in controlled laboratory settings using cells or tissues. It is important to note that these products are not categorized as medicines or drugs, and they have not received approval from the FDA for the prevention, treatment, or cure of any medical condition, ailment, or disease. We must emphasize that any form of bodily introduction of these products into humans or animals is strictly prohibited by law. It is essential to adhere to these guidelines to ensure compliance with legal and ethical standards in research and experimentation.