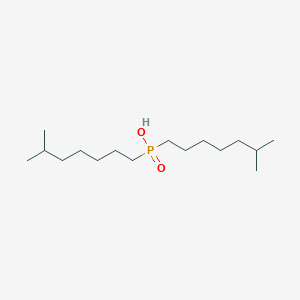
BIS(6-METHYLHEPTYL)PHOSPHINIC ACID
Overview
Description
Bis(6-methylheptyl)phosphinic acid is an organophosphorus compound characterized by two 6-methylheptyl groups attached to a phosphorus atom. Phosphinic acids (R₂POOH) are known for their moderate acidity, metal ion coordination capabilities, and utility in industrial processes such as solvent extraction and catalysis . The 6-methylheptyl substituents likely influence its solubility, steric effects, and extraction selectivity compared to other alkyl or branched derivatives.
Preparation Methods
BIS(6-METHYLHEPTYL)PHOSPHINIC ACID can be synthesized through several methods. One common synthetic route involves the reaction of sodium hypophosphite hydrate with di-tert-butyl peroxide and acetic acid at 135°C for 30 hours . The reaction mixture is then processed to obtain the desired product with a high yield and purity . Industrial production methods typically involve similar reaction conditions but on a larger scale to meet commercial demands .
Chemical Reactions Analysis
BIS(6-METHYLHEPTYL)PHOSPHINIC ACID undergoes various chemical reactions, including oxidation, reduction, and substitution reactions . Common reagents used in these reactions include oxidizing agents, reducing agents, and nucleophiles . The major products formed from these reactions depend on the specific conditions and reagents used. For example, oxidation of diisooctylphosphinic acid can lead to the formation of phosphine oxides .
Scientific Research Applications
Metal Extraction
1.1 Acidic Extractant
BIS(6-METHYLHEPTYL)PHOSPHINIC ACID is widely recognized for its effectiveness as an acidic extractant in the separation and purification of metal ions, particularly nickel and cobalt. Its ability to selectively bind to specific metal ions makes it valuable in hydrometallurgy, where it facilitates the recovery of metals from ores and waste materials.
1.2 Mechanism of Action
The extraction process typically involves the formation of metal-ligand complexes that enhance the solubility of metal ions in organic solvents. This property allows for efficient separation from aqueous solutions, making it a crucial component in processes such as solvent extraction and ion exchange.
Nanocrystal Synthesis
2.1 Role as a Ligand
In the synthesis of semiconductor nanocrystals, this compound acts as a ligand that stabilizes the nanocrystals during formation. It has been specifically noted for its role in synthesizing magic-sized cadmium selenide (CdSe) and cadmium telluride (CdTe) nanocrystals, which are essential for various optoelectronic applications.
2.2 Stabilization of Nanocrystals
The compound's ability to stabilize nanocrystals is attributed to its steric and electronic properties, which help maintain the size and shape of the particles during synthesis. This stabilization is critical for achieving desirable optical properties necessary for applications in photovoltaics and light-emitting devices.
Optoelectronic Applications
3.1 Enhancing Performance of Perovskite Devices
This compound has shown potential in enhancing the efficiency and stability of perovskite light-emitting diodes (LEDs). It facilitates ligand exchange processes that improve the integration of nanocrystals into optoelectronic devices, thus contributing to advancements in LED technology.
3.2 Room Temperature Synthesis
The compound is also involved in the room temperature synthesis of lead bromide perovskite nanocrystals, simplifying the fabrication process while maintaining high performance. This application is significant as it reduces energy consumption and increases production scalability in manufacturing processes.
Case Studies
5.1 Metal Ion Recovery
A study highlighted the use of this compound in recovering nickel from industrial effluents. The compound demonstrated a high selectivity for nickel ions over other metals, achieving a recovery rate exceeding 90% under optimized conditions.
5.2 Nanocrystal Synthesis
In another case study focused on CdSe nanocrystals, researchers reported that using this compound resulted in uniform particle sizes with enhanced photoluminescence properties compared to traditional ligands like oleic acid. This improvement underscores the compound's effectiveness in producing high-quality nanomaterials.
Mechanism of Action
The mechanism of action of diisooctylphosphinic acid involves its ability to form stable complexes with metal ions . This is achieved through the coordination of the phosphorus atom with the metal ion, leading to the formation of a stable complex . The molecular targets and pathways involved in this process depend on the specific metal ion and the conditions under which the reaction occurs .
Comparison with Similar Compounds
2.1. Structural Analogs in Solvent Extraction
Phosphinic acids are widely used as extractants for metal ions. Key analogs include:
- Cyanex 272 (bis(2,4,4-trimethylpentyl)phosphinic acid): A branched-chain phosphinic acid with high selectivity for cobalt over nickel. Its branched structure reduces steric hindrance, enhancing metal ion binding .
- D2EHPA (bis(2-ethylhexyl)phosphoric acid): A phosphoric acid (stronger acidity than phosphinic acids) with broad applications in rare earth and transition metal extraction. Phosphoric acids generally exhibit higher acidity (pKa ~1–3) compared to phosphinic acids (pKa ~3–5) due to the additional oxygen atom .
Table 1: Comparison of Organophosphorus Extractants
*Inferred from phosphinic acid class properties.
2.3. Fluorinated Phosphinic Acids
Perfluorinated derivatives (e.g., bis(tridecafluorohexyl)phosphinic acid) exhibit exceptional chemical stability and hydrophobicity due to fluorine substituents. These properties make them persistent environmental contaminants but valuable in fire-resistant materials . This compound, with non-fluorinated alkyl chains, is likely less stable under extreme conditions but more biodegradable.
2.4. Phosphonic Acid Derivatives
Phosphonates (e.g., bis(1-ethylpropyl) methylphosphonate) and phosphonic acids differ in structure (one alkyl group and an ester/acid group) and acidity. Phosphinic acids typically have lower acidity than phosphonic acids but stronger metal-binding affinity due to their dual alkyl groups .
Biological Activity
Bis(6-methylheptyl)phosphinic acid is a phosphinic acid derivative noted for its potential biological activities. This compound belongs to a class of organophosphorus compounds that have garnered interest due to their diverse applications in biochemistry, pharmacology, and environmental science. This article reviews the biological activity of this compound, including its mechanisms of action, potential therapeutic uses, and relevant case studies.
- Chemical Formula : C₁₆H₃₅O₂P
- Molecular Weight : 278.35 g/mol
- CAS Number : 13629073
Phosphinic acids, including this compound, typically function by interacting with various biological targets, such as enzymes and receptors. The compound's structure allows it to mimic substrate interactions in enzymatic reactions, particularly those involving metalloproteinases and other hydrolytic enzymes.
Enzyme Inhibition
Research indicates that phosphinic acids can serve as effective enzyme inhibitors. For example, this compound may inhibit urease activity, which is crucial in treating infections caused by urealytic bacteria like Helicobacter pylori . The inhibition mechanism often involves binding to the active site of the enzyme, thereby preventing substrate access.
Table 1: Inhibitory Activities of this compound and Analogues
Compound | Enzyme Target | IC₅₀ (µM) |
---|---|---|
This compound | Urease from Sporosarcina | 0.36 |
Aminomethyl(1-n-hexyl)phosphinic acid | Urease from Proteus mirabilis | 0.108 |
Other analogues | Various | Varies |
Case Studies and Research Findings
-
Inhibition of Ureases :
A study demonstrated that this compound effectively inhibited ureases from Sporosarcina pasteurii and Proteus mirabilis. The compound exhibited a Ki value of approximately 0.36 µM against urease from Sporosarcina, indicating strong inhibitory potential . -
Potential Therapeutic Applications :
Due to its ability to inhibit ureases, this compound is being explored as a therapeutic agent for treating infections related to urealytic bacteria. The structural modifications of phosphinic acids have shown promise in enhancing their potency and selectivity against specific bacterial strains . -
Biochemical Applications :
Phosphinic acids have been utilized in various biochemical applications, including their role as pro-drugs that convert into bioactive forms in vivo. This property enhances their therapeutic efficacy while minimizing potential side effects .
Safety and Toxicity
While this compound shows considerable biological activity, understanding its safety profile is crucial for therapeutic applications. Preliminary studies indicate low toxicity levels; however, comprehensive toxicological assessments are necessary to ensure safety for human use .
Q & A
Q. What synthetic methodologies are optimal for producing bis(6-methylheptyl)phosphinic acid with high purity, and how can reaction conditions be optimized to minimize byproducts?
Answer:
this compound can be synthesized via double amidoalkylation of hydrophosphoryl compounds, a method validated for analogous phosphinic acids . Key parameters include controlling stoichiometry (e.g., molar ratios of alkylating agents to hydrophosphoryl precursors) and reaction temperature (typically 60–80°C). Purification via column chromatography or recrystallization using non-polar solvents (e.g., hexane/ethyl acetate mixtures) is critical to isolate the product with >95% purity. Side reactions, such as incomplete alkylation or oxidation, can be mitigated by inert atmosphere conditions (N₂/Ar) and reducing agents like NaBH₄ .
Q. What advanced spectroscopic and chromatographic techniques are recommended for structural characterization of this compound?
Answer:
- Nuclear Magnetic Resonance (NMR): ¹H and ³¹P NMR are essential for confirming alkyl chain substitution patterns and phosphinic acid moiety integrity. For example, ³¹P NMR typically shows a singlet near δ 35–40 ppm for dialkylphosphinic acids .
- Mass Spectrometry (MS): High-resolution electrospray ionization (HR-ESI-MS) or MALDI-TOF can verify molecular weight and fragmentation patterns.
- Infrared Spectroscopy (IR): Peaks at 2300–2400 cm⁻¹ (P-H stretching) and 1200–1250 cm⁻¹ (P=O) confirm functional groups .
- HPLC-PDA: Reverse-phase HPLC with photodiode array detection ensures purity and identifies residual solvents or byproducts .
Q. How can researchers resolve contradictions in reported bioactivity data for this compound derivatives?
Answer:
Discrepancies in bioactivity (e.g., HIV-1 protease inhibition vs. urease inhibition) often arise from structural variations (e.g., alkyl chain length) or assay conditions . To address this:
Comparative Structure-Activity Relationship (SAR) Studies: Systematically modify alkyl substituents and evaluate inhibitory potency (e.g., IC₅₀/Kᵢ values) across enzyme targets.
Standardized Assay Protocols: Use consistent buffer pH, temperature, and enzyme sources (e.g., Sporosarcina pasteurii urease vs. recombinant HIV-1 protease) to reduce variability .
Computational Docking: Molecular dynamics simulations (e.g., AutoDock Vina) can predict binding modes and explain selectivity differences .
Q. What experimental strategies are effective for studying the competitive binding mechanism of this compound to metalloenzymes?
Answer:
For enzymes requiring metal cofactors (e.g., urease with Ni²⁺ or HIV protease with catalytic aspartates):
- Isothermal Titration Calorimetry (ITC): Quantify binding affinity (Kd) and stoichiometry by measuring heat changes during inhibitor-enzyme interactions .
- X-ray Crystallography: Resolve inhibitor-enzyme co-crystal structures to identify critical interactions (e.g., phosphinate group coordination with Ni²⁺ in urease) .
- Competitive Inhibition Assays: Use substrate analogs (e.g., urea for urease) to measure kinetic parameters (Km, Vmax) and confirm competitive vs. non-competitive modes .
Q. How can predictive toxicology models guide the safe handling of this compound in laboratory settings?
Answer:
While specific toxicity data for this compound is limited, analog studies (e.g., phenylphosphinic acid) suggest:
In Silico Tools: Use QSAR models (e.g., EPA’s TEST) to predict acute toxicity (LD₅₀) and prioritize in vitro assays .
In Vitro Cytotoxicity: Screen human cell lines (e.g., HEK293) for mitochondrial toxicity (MTT assay) and membrane integrity (LDH release).
Environmental Risk Assessment: Follow REACH guidelines to evaluate biodegradability and aquatic toxicity (e.g., Daphnia magna assays) .
Safety Protocols: Implement PPE (gloves, goggles) and fume hoods during synthesis, referencing CAS No. 121-70-0 safety sheets for analogs .
Q. What computational approaches are validated for designing this compound derivatives with enhanced enzyme inhibitory potency?
Answer:
- Molecular Docking: Screen virtual libraries of derivatives against enzyme active sites (e.g., HIV-1 protease PDB: 1HHP) to prioritize candidates with strong hydrogen bonding and hydrophobic interactions .
- Free Energy Perturbation (FEP): Calculate binding free energy differences (ΔΔG) to optimize substituent effects .
- ADMET Prediction: Tools like SwissADME forecast pharmacokinetic properties (e.g., logP, bioavailability) to balance potency and drug-likeness .
Q. How can researchers address the lack of ecological toxicity data for this compound in environmental fate studies?
Answer:
Tiered Testing Framework:
- Tier 1: Use OECD 301 biodegradability tests (e.g., modified Sturm test) to assess persistence.
- Tier 2: Conduct algal growth inhibition (OECD 201) and Daphnia acute toxicity (OECD 202) assays .
Metabolite Identification: LC-MS/MS can detect degradation products in simulated wastewater to evaluate bioaccumulation potential.
Comparative Analysis: Cross-reference data from structurally similar compounds (e.g., perfluorinated phosphinic acids) to infer environmental behavior .
Properties
IUPAC Name |
bis(6-methylheptyl)phosphinic acid | |
---|---|---|
Source | PubChem | |
URL | https://pubchem.ncbi.nlm.nih.gov | |
Description | Data deposited in or computed by PubChem | |
InChI |
InChI=1S/C16H35O2P/c1-15(2)11-7-5-9-13-19(17,18)14-10-6-8-12-16(3)4/h15-16H,5-14H2,1-4H3,(H,17,18) | |
Source | PubChem | |
URL | https://pubchem.ncbi.nlm.nih.gov | |
Description | Data deposited in or computed by PubChem | |
InChI Key |
GBNVBFGHGMAMDH-UHFFFAOYSA-N | |
Source | PubChem | |
URL | https://pubchem.ncbi.nlm.nih.gov | |
Description | Data deposited in or computed by PubChem | |
Canonical SMILES |
CC(C)CCCCCP(=O)(CCCCCC(C)C)O | |
Source | PubChem | |
URL | https://pubchem.ncbi.nlm.nih.gov | |
Description | Data deposited in or computed by PubChem | |
Molecular Formula |
C16H35O2P | |
Source | PubChem | |
URL | https://pubchem.ncbi.nlm.nih.gov | |
Description | Data deposited in or computed by PubChem | |
DSSTOX Substance ID |
DTXSID20545423 | |
Record name | Bis(6-methylheptyl)phosphinic acid | |
Source | EPA DSSTox | |
URL | https://comptox.epa.gov/dashboard/DTXSID20545423 | |
Description | DSSTox provides a high quality public chemistry resource for supporting improved predictive toxicology. | |
Molecular Weight |
290.42 g/mol | |
Source | PubChem | |
URL | https://pubchem.ncbi.nlm.nih.gov | |
Description | Data deposited in or computed by PubChem | |
CAS No. |
27661-42-3 | |
Record name | Bis(6-methylheptyl)phosphinic acid | |
Source | EPA DSSTox | |
URL | https://comptox.epa.gov/dashboard/DTXSID20545423 | |
Description | DSSTox provides a high quality public chemistry resource for supporting improved predictive toxicology. | |
Record name | Diisooctylphosphinic acid | |
Source | European Chemicals Agency (ECHA) | |
URL | https://echa.europa.eu/information-on-chemicals | |
Description | The European Chemicals Agency (ECHA) is an agency of the European Union which is the driving force among regulatory authorities in implementing the EU's groundbreaking chemicals legislation for the benefit of human health and the environment as well as for innovation and competitiveness. | |
Explanation | Use of the information, documents and data from the ECHA website is subject to the terms and conditions of this Legal Notice, and subject to other binding limitations provided for under applicable law, the information, documents and data made available on the ECHA website may be reproduced, distributed and/or used, totally or in part, for non-commercial purposes provided that ECHA is acknowledged as the source: "Source: European Chemicals Agency, http://echa.europa.eu/". Such acknowledgement must be included in each copy of the material. ECHA permits and encourages organisations and individuals to create links to the ECHA website under the following cumulative conditions: Links can only be made to webpages that provide a link to the Legal Notice page. | |
Retrosynthesis Analysis
AI-Powered Synthesis Planning: Our tool employs the Template_relevance Pistachio, Template_relevance Bkms_metabolic, Template_relevance Pistachio_ringbreaker, Template_relevance Reaxys, Template_relevance Reaxys_biocatalysis model, leveraging a vast database of chemical reactions to predict feasible synthetic routes.
One-Step Synthesis Focus: Specifically designed for one-step synthesis, it provides concise and direct routes for your target compounds, streamlining the synthesis process.
Accurate Predictions: Utilizing the extensive PISTACHIO, BKMS_METABOLIC, PISTACHIO_RINGBREAKER, REAXYS, REAXYS_BIOCATALYSIS database, our tool offers high-accuracy predictions, reflecting the latest in chemical research and data.
Strategy Settings
Precursor scoring | Relevance Heuristic |
---|---|
Min. plausibility | 0.01 |
Model | Template_relevance |
Template Set | Pistachio/Bkms_metabolic/Pistachio_ringbreaker/Reaxys/Reaxys_biocatalysis |
Top-N result to add to graph | 6 |
Feasible Synthetic Routes
Disclaimer and Information on In-Vitro Research Products
Please be aware that all articles and product information presented on BenchChem are intended solely for informational purposes. The products available for purchase on BenchChem are specifically designed for in-vitro studies, which are conducted outside of living organisms. In-vitro studies, derived from the Latin term "in glass," involve experiments performed in controlled laboratory settings using cells or tissues. It is important to note that these products are not categorized as medicines or drugs, and they have not received approval from the FDA for the prevention, treatment, or cure of any medical condition, ailment, or disease. We must emphasize that any form of bodily introduction of these products into humans or animals is strictly prohibited by law. It is essential to adhere to these guidelines to ensure compliance with legal and ethical standards in research and experimentation.