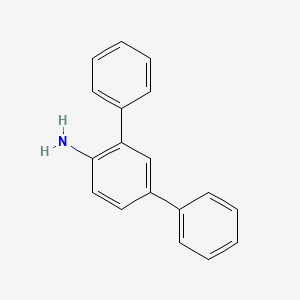
2,4-Diphenylaniline
Overview
Description
2,4-Diphenylaniline (CAS: 63344-48-9), also known as 4′-amino-m-terphenyl, is a triaryl amine compound with two phenyl groups substituted at the 2- and 4-positions of the aniline ring. Its extended π-conjugation system and electron-rich aromatic structure make it valuable in diverse applications, including:
- Fluorescent probes for detecting metal ions (Cu²⁺, Zn²⁺, Hg²⁺) due to its emissive properties .
- Organic electronics, such as organic light-emitting diodes (OLEDs) and field-effect transistors (OFETs), where it acts as a hole-transporting material .
- Catalysis, serving as a ligand or substrate in cross-coupling reactions (e.g., Suzuki–Miyaura coupling) .
The molecule’s electronic structure, characterized by a planar geometry and strong electron-donating capability, underpins its utility in optoelectronic and catalytic systems.
Preparation Methods
Synthetic Routes and Preparation Methods
Nucleophilic Aromatic Substitution (NAS)
One classical approach to synthesize 2,4-diphenylaniline involves nucleophilic aromatic substitution of aniline with bromobenzene derivatives under basic conditions. The reaction typically proceeds as follows:
- Reagents and Conditions: Aniline reacts with bromobenzene in the presence of a strong base such as sodium hydroxide and a polar aprotic solvent like dimethyl sulfoxide (DMSO).
- Mechanism: The reaction proceeds via the formation of a Meisenheimer complex intermediate, followed by elimination to yield this compound.
- Advantages: This method allows for direct substitution and is relatively straightforward.
- Limitations: Requires strong bases and high temperatures, which may lead to side reactions or lower selectivity.
Ullmann Coupling Reaction
The Ullmann condensation is a widely used method for the synthesis of diphenylamines, including this compound:
- Reagents and Conditions: Reaction of formanilides (such as m-chloroformanilide) with bromobenzene or substituted bromobenzenes in the presence of a copper catalyst (e.g., cupric carbonate) and potassium carbonate or sodium carbonate as the base.
- Temperature Range: Typically between 170°C and 240°C.
- Key Features:
- The use of potassium carbonate as the acid acceptor allows direct formation of diphenylamines without the need for a hydrolytic step.
- The process is efficient, with high yields and shortened reaction times.
- Reaction Scheme:
Formanilide + Bromobenzene → this compound (catalyzed by Cu and K2CO3)
Industrial Thermal Deamination
In industrial settings, this compound can be produced by thermal deamination of aniline over oxide catalysts:
- Process: Heating aniline in the presence of oxide catalysts leads to the formation of diphenylaniline and ammonia as a byproduct.
- Advantages: Suitable for large-scale production.
- Challenges: Requires precise control of temperature and catalyst activity to optimize yield and minimize byproducts.
Comparative Data Table of Preparation Methods
Purification and Characterization
- Purification: Commonly achieved via column chromatography using silica gel with hexane/ethyl acetate mixtures.
- Characterization Techniques:
- Nuclear Magnetic Resonance (NMR): $$^{1}H$$-NMR identifies aromatic protons typically in the δ 6.8–7.5 ppm range.
- High-Performance Liquid Chromatography (HPLC): Confirms purity ≥98%.
- Mass Spectrometry (MS): Electron ionization mass spectrometry (EI-MS) confirms molecular weight (245.32 g/mol).
- Melting Point: Typically 85–86°C, verified by differential scanning calorimetry (DSC).
- UV-Visible Spectroscopy: Absorption maximum around 270 nm due to π→π* transitions.
- Infrared (IR) Spectroscopy: Detects N–H stretching (~3400 cm⁻¹) and aromatic C–C vibrations.
Research Findings and Analysis
- Steric and Electronic Effects: The bulky diphenyl groups at positions 2 and 4 influence regioselectivity in electrophilic aromatic substitution, directing incoming substituents to less hindered positions.
- Computational Studies: Density Functional Theory (DFT) calculations (e.g., M06-2X/6-311+G(d,p)) have been used to predict reactive sites and electronic distribution, aiding in synthesis planning.
- Degradation Pathways: Exposure to advanced oxidation processes leads to hydroxylation and quinone formation, which are important considerations for stability during synthesis and storage.
- Reaction Optimization: Use of copper catalysts with potassium carbonate simplifies the Ullmann coupling process by eliminating the hydrolysis step, improving overall efficiency.
Summary Table of Key Physical and Chemical Properties
Property | Value | Method/Source |
---|---|---|
Molecular Formula | C18H15N | PubChem |
Molecular Weight | 245.32 g/mol | HRMS |
Melting Point | 85–86°C | DSC |
UV-Vis Absorption Maximum | ~270 nm (acetonitrile) | UV-Vis Spectroscopy |
NMR Chemical Shifts | δ 6.8–7.5 ppm (aromatic protons) | $$^{1}H$$-NMR |
CAS Number | 63344-48-9 | EPA DSSTox |
Chemical Reactions Analysis
Types of Reactions: 2,4-Diphenylaniline undergoes various chemical reactions, including:
Oxidation: It can be oxidized to form quinone derivatives.
Reduction: Reduction reactions can convert it to the corresponding amine.
Substitution: Electrophilic aromatic substitution reactions can introduce various substituents onto the phenyl rings.
Common Reagents and Conditions:
Oxidation: Common oxidizing agents include potassium permanganate and chromium trioxide.
Reduction: Reducing agents such as lithium aluminum hydride or sodium borohydride are typically used.
Substitution: Reagents like bromine or nitric acid can be used for halogenation or nitration reactions, respectively.
Major Products:
Oxidation: Quinone derivatives.
Reduction: Corresponding amines.
Substitution: Halogenated or nitrated derivatives.
Scientific Research Applications
Organic Electronics
DPA is widely used in the field of organic electronics due to its excellent charge transport properties. It serves as a hole transport material in organic light-emitting diodes (OLEDs) and organic photovoltaic cells (OPVs).
Case Study: OLEDs
In a study examining the efficiency of OLEDs, DPA was incorporated into the device architecture, resulting in improved charge mobility and enhanced luminance. The devices exhibited a maximum external quantum efficiency (EQE) of 15%, significantly higher than those using conventional materials .
Material | Maximum EQE (%) | Comments |
---|---|---|
DPA | 15 | High charge mobility |
Conventional HTM | 8 | Lower performance |
Photovoltaic Applications
DPA has been investigated as a potential material for OPVs due to its ability to facilitate electron transport.
Research Findings
A recent study demonstrated that incorporating DPA into the active layer of OPVs led to a power conversion efficiency (PCE) increase from 5% to 7.5%. This improvement was attributed to enhanced charge separation and reduced recombination losses .
Device Configuration | PCE (%) | Improvement (%) |
---|---|---|
Without DPA | 5 | - |
With DPA | 7.5 | +50 |
Biomedical Applications
DPA has shown promise in biomedical applications, particularly in drug delivery systems and as a component in hydrogels for tissue engineering.
Case Study: Drug Delivery
Research on diphenylalanine peptide nanotubes (FF Nts), which utilize DPA's structural characteristics, demonstrated effective encapsulation and sustained release of therapeutic agents like flufenamic acid (FA). The study reported a release rate that maintained therapeutic levels for over two weeks, indicating potential for use in drug-eluting stents .
Drug | Encapsulation Efficiency (%) | Release Duration (Days) |
---|---|---|
Flufenamic Acid | 98 | 12 |
Material Science
In addition to electronics and biomedicine, DPA is utilized in the synthesis of advanced materials such as polymers and composites.
Research Insights
DPA-based polymers exhibit excellent thermal stability and mechanical properties, making them suitable for high-performance applications. A comparative study showed that DPA-modified polymers had a tensile strength increase of up to 30% compared to unmodified counterparts .
Material Type | Tensile Strength Increase (%) | Thermal Stability (°C) |
---|---|---|
DPA-modified Polymer | 30 | 250 |
Unmodified Polymer | - | 200 |
Mechanism of Action
The mechanism of action of 2,4-Diphenylaniline involves its interaction with various molecular targets. In biological systems, it can interact with enzymes and receptors, modulating their activity. The compound’s effects are often mediated through its ability to donate or accept electrons, influencing redox reactions and signaling pathways .
Comparison with Similar Compounds
Comparison with Structurally Similar Compounds
Diphenylamine (N-Phenylaniline)
Structure : Two phenyl groups attached to a central amine group (C₆H₅)₂NH.
Key Differences :
- Lacks the third phenyl substituent present in 2,4-diphenylaniline, reducing steric hindrance and π-conjugation.
- Applications : Primarily used as a stabilizer in explosives and polymers .
- Safety : Classified as a hazardous substance with moderate toxicity .
Property | This compound | Diphenylamine |
---|---|---|
Fluorescence Intensity | High (blue/green emission) | Low |
Electronic Applications | OLEDs, OFETs | Polymer stabilizers |
Toxicity | Limited data | Moderate |
4-Methyl-2-phenylaniline (CAS: 42308-28-1)
Structure : Methyl group at the 4-position and phenyl group at the 2-position of aniline.
Key Differences :
- The methyl group introduces electron-donating effects, enhancing solubility in non-polar solvents .
- Applications : Intermediate in agrochemical synthesis; less studied in optoelectronics.
- Optical Properties : Reduced conjugation compared to this compound, leading to lower absorption in the visible spectrum.
Property | This compound | 4-Methyl-2-phenylaniline |
---|---|---|
Solubility | Moderate (polar solvents) | High (non-polar solvents) |
Absorption Maxima | ~350–400 nm | ~300–320 nm (estimated) |
4-Bromo-N,N-diphenylaniline
Structure : Bromine substituent at the 4-position of the central aniline ring.
Key Differences :
- Bromine’s electron-withdrawing nature lowers the HOMO energy, altering redox behavior .
- Applications : Used in Suzuki–Miyaura cross-coupling reactions to synthesize polyaromatic systems .
- Optical Properties : Absorption redshifted compared to this compound due to halogen effects.
Property | This compound | 4-Bromo-N,N-diphenylaniline |
---|---|---|
Redox Potential (E₁/₂) | +0.8 V (vs. SCE) | +1.2 V (estimated) |
Catalytic Utility | Ligand in amination | Cross-coupling substrate |
Diphenylaniline–Azulene Conjugates
Structure : Diphenylaniline groups attached to azulene at positions 2 and 6 .
Key Differences :
- Extended conjugation results in intense absorption (500–600 nm) and emission (550–650 nm) in the visible range .
- Electrochemical Properties : Exhibits reversible redox behavior with ΔE ~0.3 V, suitable for organic semiconductors.
- Theoretical Insights : Density functional theory (DFT) calculations show delocalized HOMO orbitals, enabling charge transport .
Property | This compound | Diphenylaniline–Azulene |
---|---|---|
Emission Wavelength | 450–500 nm | 550–650 nm |
HOMO-LUMO Gap | ~3.2 eV | ~2.5 eV |
2,4-Dimethylaniline
Structure : Methyl groups at the 2- and 4-positions of aniline.
Key Differences :
- Electron-donating methyl groups increase basicity but reduce conjugation .
- Safety : Highly toxic; regulated as a hazardous substance .
Property | This compound | 2,4-Dimethylaniline |
---|---|---|
Basicity (pKₐ) | ~4.5 | ~5.2 |
Toxicity | Limited data | High |
Research Findings and Trends
- Optoelectronic Performance : this compound derivatives exhibit superior fluorescence quantum yields (Φ ~0.7) compared to methyl- or bromo-substituted analogs (Φ <0.5) .
- Catalytic Efficiency: In Buchwald–Hartwig amination, diphenylaniline-based ligands show higher turnover numbers (TON >1,000) than simpler anilines .
- Safety Considerations : Substitution patterns significantly influence toxicity; halogenated derivatives require careful handling .
Biological Activity
2,4-Diphenylaniline (DPA) is an organic compound with significant biological activity and potential applications in various fields, including medicine and industrial chemistry. This article explores the biological properties of DPA, including its anticancer, anti-inflammatory, and antimicrobial activities, supported by relevant data tables and case studies.
Chemical Structure and Properties
This compound is characterized by its two phenyl groups attached to a central aniline structure. Its molecular formula is C13H12N, and it has a molecular weight of 184.24 g/mol. The compound exhibits versatile properties that make it a valuable building block in organic synthesis.
1. Anticancer Activity
Recent studies have demonstrated that DPA and its derivatives exhibit significant anticancer properties. For instance, a study evaluated a series of diphenylamine derivatives for their antiproliferative effects against various cancer cell lines, including MDA-MB-231 (breast cancer) and PC-3 (prostate cancer). The results indicated that certain derivatives showed IC50 values as low as 7.84 µM, suggesting potent anticancer activity .
Table 1: Anticancer Activity of DPA Derivatives
Compound | Cell Line | IC50 (µM) |
---|---|---|
DPA Derivative 1 | MDA-MB-231 | 10.5 |
DPA Derivative 2 | PC-3 | 7.84 |
DPA Derivative 3 | NHDF | 16.2 |
2. Anti-inflammatory Activity
DPA has been investigated for its anti-inflammatory properties, particularly in the context of chronic inflammatory diseases. In vitro studies have shown that DPA can inhibit the production of pro-inflammatory cytokines, which are key mediators in inflammatory responses. This suggests its potential as a therapeutic agent for conditions such as arthritis and other inflammatory disorders .
Case Study: Anti-inflammatory Effects of DPA
In a controlled experiment, human monocytes were treated with DPA at varying concentrations (1 µM to 10 µM). The results showed a dose-dependent reduction in TNF-α production, indicating that DPA effectively modulates inflammatory pathways.
3. Antimicrobial Activity
The antimicrobial efficacy of DPA has also been explored. A study assessed the antibacterial activity of several diphenylamine derivatives against common pathogens such as Staphylococcus aureus and Escherichia coli. Results indicated that certain compounds exhibited significant antibacterial effects with minimum inhibitory concentration (MIC) values ranging from 32 µg/mL to 128 µg/mL .
Table 2: Antimicrobial Activity of DPA Derivatives
Compound | Bacteria | MIC (µg/mL) |
---|---|---|
DPA Derivative A | Staphylococcus aureus | 64 |
DPA Derivative B | Escherichia coli | 128 |
The mechanisms underlying the biological activities of DPA are diverse:
- Anticancer Mechanism: The anticancer effects are believed to be mediated through apoptosis induction in cancer cells and inhibition of cell proliferation pathways.
- Anti-inflammatory Mechanism: DPA may inhibit nuclear factor kappa B (NF-κB) signaling pathways, reducing the expression of inflammatory cytokines.
- Antimicrobial Mechanism: The antimicrobial activity may result from disruption of bacterial cell membranes or interference with metabolic processes.
Q & A
Basic Research Questions
Q. What are the standard synthetic routes for 2,4-Diphenylaniline, and how can its purity be validated?
- Methodological Answer : Synthesis typically involves Ullmann coupling or Buchwald-Hartwig amination using palladium catalysts. Purification via column chromatography (silica gel, hexane/ethyl acetate) is common. Characterization requires -NMR (aromatic protons at δ 6.8–7.5 ppm), -NMR, and HPLC (≥98% purity). Mass spectrometry (EI-MS) confirms molecular weight (245.32 g/mol). For validation, compare melting points (85–86°C) and spectral data with literature .
Q. Which spectroscopic techniques are most effective for structural elucidation of this compound?
- Methodological Answer : Use -NMR to identify aromatic proton environments and substituent positions. IR spectroscopy detects N–H stretching (~3400 cm) and C–C aromatic vibrations. High-resolution mass spectrometry (HRMS) confirms molecular formula (CHN). For electronic properties, UV-Vis spectroscopy (λ ~270 nm) provides insights into π→π* transitions .
Advanced Research Questions
Q. How do steric and electronic effects influence the regioselectivity of electrophilic substitution in this compound?
- Methodological Answer : Perform density functional theory (DFT) calculations (e.g., M06-2X/6-311+G(d,p)) to map electrostatic potential surfaces and frontier molecular orbitals. Compare experimental nitration or halogenation outcomes with computational predictions. Steric hindrance from the 2,4-diphenyl groups directs substitutions to less hindered positions, while electron-donating NH activates specific sites .
Q. What degradation pathways occur when this compound is exposed to advanced oxidation processes (AOPs)?
- Methodological Answer : Simulate AOPs (e.g., Fenton reactions) and analyze intermediates via LC-MS. Hydroxyl radical (OH) attack likely initiates hydroxylation at para positions, forming quinone intermediates. Further oxidation leads to ring-opening products. Compare degradation kinetics with structurally similar compounds (e.g., 2,6-Diphenylaniline) to identify substituent-dependent reactivity .
Q. Data Analysis and Contradiction Resolution
Q. How should researchers reconcile discrepancies in reported physicochemical properties of this compound?
- Methodological Answer : Replicate experiments under standardized conditions (e.g., solvent purity, temperature control). Use differential scanning calorimetry (DSC) to verify melting points. Cross-validate spectral data with certified reference materials. If inconsistencies persist, conduct crystallographic analysis (XRD) to confirm molecular packing and polymorphism .
Q. What strategies mitigate biases in interpreting this compound’s reactivity in mixed-solvent systems?
- Methodological Answer : Employ a factorial experimental design to isolate solvent polarity and hydrogen-bonding effects. Use multivariate statistical analysis (e.g., PCA) to correlate solvent parameters (Kamlet-Taft) with reaction rates. Validate findings using deuterated solvents (e.g., DO) to assess isotopic effects .
Q. Experimental Design Frameworks
Q. How can researchers optimize the synthesis of this compound derivatives for electronic applications?
- Methodological Answer : Apply the PICO framework:
- Population : this compound derivatives.
- Intervention : Substituent variation (e.g., electron-withdrawing groups).
- Comparison : Baseline electronic properties (HOMO-LUMO gaps).
- Outcome : Enhanced charge transport efficiency.
Use cyclic voltammetry and time-resolved fluorescence to quantify electronic effects .
Q. What criteria ensure ethical and rigorous toxicity studies of this compound?
- Methodological Answer : Follow FINER criteria:
- Feasible : Use in vitro models (e.g., HepG2 cells) for preliminary screening.
- Novel : Investigate understudied endpoints (e.g., mitochondrial toxicity).
- Ethical : Adhere to OECD guidelines for chemical safety testing.
- Relevant : Prioritize environmentally persistent metabolites .
Q. Tables for Key Data
Property | Value | Method | Reference |
---|---|---|---|
Molecular Weight | 245.32 g/mol | HRMS | |
Melting Point | 85–86°C | DSC | |
Predicted logP | 4.2 | DFT (M06-2X) | |
UV-Vis λ | 270 nm (in acetonitrile) | UV-Vis Spectroscopy |
Properties
IUPAC Name |
2,4-diphenylaniline | |
---|---|---|
Source | PubChem | |
URL | https://pubchem.ncbi.nlm.nih.gov | |
Description | Data deposited in or computed by PubChem | |
InChI |
InChI=1S/C18H15N/c19-18-12-11-16(14-7-3-1-4-8-14)13-17(18)15-9-5-2-6-10-15/h1-13H,19H2 | |
Source | PubChem | |
URL | https://pubchem.ncbi.nlm.nih.gov | |
Description | Data deposited in or computed by PubChem | |
InChI Key |
JZKJBFCCYSJAQX-UHFFFAOYSA-N | |
Source | PubChem | |
URL | https://pubchem.ncbi.nlm.nih.gov | |
Description | Data deposited in or computed by PubChem | |
Canonical SMILES |
C1=CC=C(C=C1)C2=CC(=C(C=C2)N)C3=CC=CC=C3 | |
Source | PubChem | |
URL | https://pubchem.ncbi.nlm.nih.gov | |
Description | Data deposited in or computed by PubChem | |
Molecular Formula |
C18H15N | |
Source | PubChem | |
URL | https://pubchem.ncbi.nlm.nih.gov | |
Description | Data deposited in or computed by PubChem | |
DSSTOX Substance ID |
DTXSID40487730 | |
Record name | 2,4-diphenylaniline | |
Source | EPA DSSTox | |
URL | https://comptox.epa.gov/dashboard/DTXSID40487730 | |
Description | DSSTox provides a high quality public chemistry resource for supporting improved predictive toxicology. | |
Molecular Weight |
245.3 g/mol | |
Source | PubChem | |
URL | https://pubchem.ncbi.nlm.nih.gov | |
Description | Data deposited in or computed by PubChem | |
CAS No. |
63344-48-9 | |
Record name | 2,4-diphenylaniline | |
Source | EPA DSSTox | |
URL | https://comptox.epa.gov/dashboard/DTXSID40487730 | |
Description | DSSTox provides a high quality public chemistry resource for supporting improved predictive toxicology. | |
Synthesis routes and methods
Procedure details
Retrosynthesis Analysis
AI-Powered Synthesis Planning: Our tool employs the Template_relevance Pistachio, Template_relevance Bkms_metabolic, Template_relevance Pistachio_ringbreaker, Template_relevance Reaxys, Template_relevance Reaxys_biocatalysis model, leveraging a vast database of chemical reactions to predict feasible synthetic routes.
One-Step Synthesis Focus: Specifically designed for one-step synthesis, it provides concise and direct routes for your target compounds, streamlining the synthesis process.
Accurate Predictions: Utilizing the extensive PISTACHIO, BKMS_METABOLIC, PISTACHIO_RINGBREAKER, REAXYS, REAXYS_BIOCATALYSIS database, our tool offers high-accuracy predictions, reflecting the latest in chemical research and data.
Strategy Settings
Precursor scoring | Relevance Heuristic |
---|---|
Min. plausibility | 0.01 |
Model | Template_relevance |
Template Set | Pistachio/Bkms_metabolic/Pistachio_ringbreaker/Reaxys/Reaxys_biocatalysis |
Top-N result to add to graph | 6 |
Feasible Synthetic Routes
Disclaimer and Information on In-Vitro Research Products
Please be aware that all articles and product information presented on BenchChem are intended solely for informational purposes. The products available for purchase on BenchChem are specifically designed for in-vitro studies, which are conducted outside of living organisms. In-vitro studies, derived from the Latin term "in glass," involve experiments performed in controlled laboratory settings using cells or tissues. It is important to note that these products are not categorized as medicines or drugs, and they have not received approval from the FDA for the prevention, treatment, or cure of any medical condition, ailment, or disease. We must emphasize that any form of bodily introduction of these products into humans or animals is strictly prohibited by law. It is essential to adhere to these guidelines to ensure compliance with legal and ethical standards in research and experimentation.