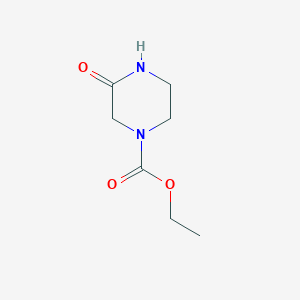
Ethyl 3-oxopiperazine-1-carboxylate
Overview
Description
Ethyl 3-oxopiperazine-1-carboxylate is a chemical compound with the molecular formula C7H12N2O3 . It is a derivative of N-Boc piperazine .
Synthesis Analysis
The synthesis of Ethyl 3-oxopiperazine-1-carboxylate involves the reaction of (3-oxopiperazine-2-ylidene)ethanoates with 2-bromo-1,1-diethoxyethane . Two derivatives of N-Boc piperazine, an ester derivative, i.e., tert-butyl 4- (2-ethoxy-2-oxoethyl)-piperazine-1-carboxylate (1), and, a hydrazide derivative tert-butyl 4- (2-hydrazino-2-oxoethyl)piperazine-1-carboxylate (2) were synthesized and were characterized by FT-IR, 1 H & 13 C NMR and LCMS spectroscopic studies .Molecular Structure Analysis
The molecular weight of Ethyl 3-oxopiperazine-1-carboxylate is 172.18 . The molecule of 1 is linear in shape with the ethyl acetate moiety adopting fully extended conformation, while the molecule of 2 is L-shaped with the molecule being twisted at the C10 atom .Chemical Reactions Analysis
The chemical reactions involving Ethyl 3-oxopiperazine-1-carboxylate are not sensitive to the substituent effect of salicylaldehyde . A range of electron-withdrawing substituents at different positions on the ring tolerated reaction conditions well enough to furnish corresponding products in good to excellent yields .Physical And Chemical Properties Analysis
Ethyl 3-oxopiperazine-1-carboxylate is a solid substance . Its melting point is 118-122 °C .Mechanism of Action
Safety and Hazards
Future Directions
Ethyl 3-oxopiperazine-1-carboxylate and its derivatives serve as useful building blocks/intermediates in the synthesis of several novel organic compounds such as amides, sulphonamides, Mannich bases, Schiff’s bases, thiazolidinones, azetidinones, and imidazolinones . These derived compounds have shown a wide spectrum of biological activities such as the antibacterial, antifungal, anticancer, antiparasitic, antihistamine, and antidepressive activities .
properties
IUPAC Name |
ethyl 3-oxopiperazine-1-carboxylate | |
---|---|---|
Source | PubChem | |
URL | https://pubchem.ncbi.nlm.nih.gov | |
Description | Data deposited in or computed by PubChem | |
InChI |
InChI=1S/C7H12N2O3/c1-2-12-7(11)9-4-3-8-6(10)5-9/h2-5H2,1H3,(H,8,10) | |
Source | PubChem | |
URL | https://pubchem.ncbi.nlm.nih.gov | |
Description | Data deposited in or computed by PubChem | |
InChI Key |
CWVCSOZZZJTENQ-UHFFFAOYSA-N | |
Source | PubChem | |
URL | https://pubchem.ncbi.nlm.nih.gov | |
Description | Data deposited in or computed by PubChem | |
Canonical SMILES |
CCOC(=O)N1CCNC(=O)C1 | |
Source | PubChem | |
URL | https://pubchem.ncbi.nlm.nih.gov | |
Description | Data deposited in or computed by PubChem | |
Molecular Formula |
C7H12N2O3 | |
Source | PubChem | |
URL | https://pubchem.ncbi.nlm.nih.gov | |
Description | Data deposited in or computed by PubChem | |
DSSTOX Substance ID |
DTXSID40525969 | |
Record name | Ethyl 3-oxopiperazine-1-carboxylate | |
Source | EPA DSSTox | |
URL | https://comptox.epa.gov/dashboard/DTXSID40525969 | |
Description | DSSTox provides a high quality public chemistry resource for supporting improved predictive toxicology. | |
Molecular Weight |
172.18 g/mol | |
Source | PubChem | |
URL | https://pubchem.ncbi.nlm.nih.gov | |
Description | Data deposited in or computed by PubChem | |
Product Name |
Ethyl 3-oxopiperazine-1-carboxylate | |
CAS RN |
59701-99-4 | |
Record name | Ethyl 3-oxopiperazine-1-carboxylate | |
Source | EPA DSSTox | |
URL | https://comptox.epa.gov/dashboard/DTXSID40525969 | |
Description | DSSTox provides a high quality public chemistry resource for supporting improved predictive toxicology. | |
Synthesis routes and methods
Procedure details
Retrosynthesis Analysis
AI-Powered Synthesis Planning: Our tool employs the Template_relevance Pistachio, Template_relevance Bkms_metabolic, Template_relevance Pistachio_ringbreaker, Template_relevance Reaxys, Template_relevance Reaxys_biocatalysis model, leveraging a vast database of chemical reactions to predict feasible synthetic routes.
One-Step Synthesis Focus: Specifically designed for one-step synthesis, it provides concise and direct routes for your target compounds, streamlining the synthesis process.
Accurate Predictions: Utilizing the extensive PISTACHIO, BKMS_METABOLIC, PISTACHIO_RINGBREAKER, REAXYS, REAXYS_BIOCATALYSIS database, our tool offers high-accuracy predictions, reflecting the latest in chemical research and data.
Strategy Settings
Precursor scoring | Relevance Heuristic |
---|---|
Min. plausibility | 0.01 |
Model | Template_relevance |
Template Set | Pistachio/Bkms_metabolic/Pistachio_ringbreaker/Reaxys/Reaxys_biocatalysis |
Top-N result to add to graph | 6 |
Feasible Synthetic Routes
Disclaimer and Information on In-Vitro Research Products
Please be aware that all articles and product information presented on BenchChem are intended solely for informational purposes. The products available for purchase on BenchChem are specifically designed for in-vitro studies, which are conducted outside of living organisms. In-vitro studies, derived from the Latin term "in glass," involve experiments performed in controlled laboratory settings using cells or tissues. It is important to note that these products are not categorized as medicines or drugs, and they have not received approval from the FDA for the prevention, treatment, or cure of any medical condition, ailment, or disease. We must emphasize that any form of bodily introduction of these products into humans or animals is strictly prohibited by law. It is essential to adhere to these guidelines to ensure compliance with legal and ethical standards in research and experimentation.