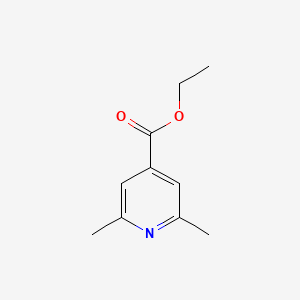
Ethyl 2,6-dimethylisonicotinate
Overview
Description
Ethyl 2,6-dimethylisonicotinate is a chemical compound that belongs to the class of isonicotinic acid derivatives. It is a yellowish crystalline powder that is soluble in organic solvents. The molecular formula of this compound is C10H13NO2, and it has a molecular weight of 179.22 g/mol . This compound has been studied for its potential therapeutic and environmental applications.
Preparation Methods
Synthetic Routes and Reaction Conditions
Ethyl 2,6-dimethylisonicotinate can be synthesized through various organic synthesis methods. One common method involves the esterification of 2,6-dimethylisonicotinic acid with ethanol in the presence of a catalyst such as sulfuric acid. The reaction is typically carried out under reflux conditions to ensure complete conversion of the acid to the ester .
Industrial Production Methods
In industrial settings, the production of this compound may involve continuous flow processes to enhance efficiency and yield. The use of automated reactors and precise control of reaction parameters such as temperature, pressure, and reactant concentrations can lead to higher purity and consistent quality of the final product .
Chemical Reactions Analysis
Types of Reactions
Ethyl 2,6-dimethylisonicotinate undergoes various chemical reactions, including:
Oxidation: This compound can be oxidized to form corresponding carboxylic acids or other oxidized derivatives.
Reduction: Reduction reactions can convert this compound to its corresponding alcohols or amines.
Substitution: It can undergo nucleophilic substitution reactions, where the ester group is replaced by other functional groups.
Common Reagents and Conditions
Oxidation: Common oxidizing agents include potassium permanganate (KMnO4) and chromium trioxide (CrO3).
Reduction: Reducing agents such as lithium aluminum hydride (LiAlH4) and sodium borohydride (NaBH4) are often used.
Substitution: Nucleophiles like amines, alcohols, and thiols can be used under basic or acidic conditions to facilitate substitution reactions.
Major Products Formed
The major products formed from these reactions depend on the specific reagents and conditions used. For example, oxidation may yield carboxylic acids, while reduction can produce alcohols or amines .
Scientific Research Applications
Ethyl 2,6-dimethylisonicotinate has a wide range of scientific research applications, including:
Chemistry: It is used as a building block in the synthesis of more complex organic molecules and as a reagent in various organic reactions.
Biology: This compound has been studied for its potential biological activities, including antimicrobial and antiviral properties.
Medicine: Research has explored its potential therapeutic applications, such as in the development of new drugs for treating various diseases.
Industry: this compound is used in the production of agrochemicals, pharmaceuticals, and other industrial chemicals.
Mechanism of Action
The mechanism of action of Ethyl 2,6-dimethylisonicotinate involves its interaction with specific molecular targets and pathways. For instance, it may act as an inhibitor of certain enzymes or receptors, thereby modulating biochemical pathways involved in disease processes . The exact molecular targets and pathways can vary depending on the specific application and context of use .
Comparison with Similar Compounds
Similar Compounds
Methyl 2,6-dimethylisonicotinate: Similar in structure but with a methyl ester group instead of an ethyl ester group.
2,6-Dichloroisonicotinic acid: Contains chlorine atoms instead of methyl groups, leading to different chemical properties and applications.
Uniqueness
This compound is unique due to its specific ester group and methyl substitutions, which confer distinct chemical and biological properties.
Properties
IUPAC Name |
ethyl 2,6-dimethylpyridine-4-carboxylate | |
---|---|---|
Source | PubChem | |
URL | https://pubchem.ncbi.nlm.nih.gov | |
Description | Data deposited in or computed by PubChem | |
InChI |
InChI=1S/C10H13NO2/c1-4-13-10(12)9-5-7(2)11-8(3)6-9/h5-6H,4H2,1-3H3 | |
Source | PubChem | |
URL | https://pubchem.ncbi.nlm.nih.gov | |
Description | Data deposited in or computed by PubChem | |
InChI Key |
HUKOOYGGHKWWDG-UHFFFAOYSA-N | |
Source | PubChem | |
URL | https://pubchem.ncbi.nlm.nih.gov | |
Description | Data deposited in or computed by PubChem | |
Canonical SMILES |
CCOC(=O)C1=CC(=NC(=C1)C)C | |
Source | PubChem | |
URL | https://pubchem.ncbi.nlm.nih.gov | |
Description | Data deposited in or computed by PubChem | |
Molecular Formula |
C10H13NO2 | |
Source | PubChem | |
URL | https://pubchem.ncbi.nlm.nih.gov | |
Description | Data deposited in or computed by PubChem | |
DSSTOX Substance ID |
DTXSID90482098 | |
Record name | Ethyl 2,6-dimethylpyridine-4-carboxylate | |
Source | EPA DSSTox | |
URL | https://comptox.epa.gov/dashboard/DTXSID90482098 | |
Description | DSSTox provides a high quality public chemistry resource for supporting improved predictive toxicology. | |
Molecular Weight |
179.22 g/mol | |
Source | PubChem | |
URL | https://pubchem.ncbi.nlm.nih.gov | |
Description | Data deposited in or computed by PubChem | |
CAS No. |
39965-80-5 | |
Record name | Ethyl 2,6-dimethylpyridine-4-carboxylate | |
Source | EPA DSSTox | |
URL | https://comptox.epa.gov/dashboard/DTXSID90482098 | |
Description | DSSTox provides a high quality public chemistry resource for supporting improved predictive toxicology. | |
Retrosynthesis Analysis
AI-Powered Synthesis Planning: Our tool employs the Template_relevance Pistachio, Template_relevance Bkms_metabolic, Template_relevance Pistachio_ringbreaker, Template_relevance Reaxys, Template_relevance Reaxys_biocatalysis model, leveraging a vast database of chemical reactions to predict feasible synthetic routes.
One-Step Synthesis Focus: Specifically designed for one-step synthesis, it provides concise and direct routes for your target compounds, streamlining the synthesis process.
Accurate Predictions: Utilizing the extensive PISTACHIO, BKMS_METABOLIC, PISTACHIO_RINGBREAKER, REAXYS, REAXYS_BIOCATALYSIS database, our tool offers high-accuracy predictions, reflecting the latest in chemical research and data.
Strategy Settings
Precursor scoring | Relevance Heuristic |
---|---|
Min. plausibility | 0.01 |
Model | Template_relevance |
Template Set | Pistachio/Bkms_metabolic/Pistachio_ringbreaker/Reaxys/Reaxys_biocatalysis |
Top-N result to add to graph | 6 |
Feasible Synthetic Routes
Disclaimer and Information on In-Vitro Research Products
Please be aware that all articles and product information presented on BenchChem are intended solely for informational purposes. The products available for purchase on BenchChem are specifically designed for in-vitro studies, which are conducted outside of living organisms. In-vitro studies, derived from the Latin term "in glass," involve experiments performed in controlled laboratory settings using cells or tissues. It is important to note that these products are not categorized as medicines or drugs, and they have not received approval from the FDA for the prevention, treatment, or cure of any medical condition, ailment, or disease. We must emphasize that any form of bodily introduction of these products into humans or animals is strictly prohibited by law. It is essential to adhere to these guidelines to ensure compliance with legal and ethical standards in research and experimentation.