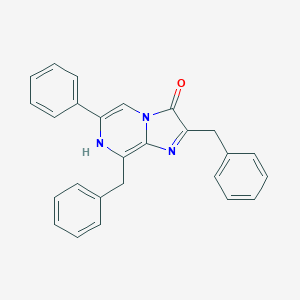
Coelenterazine 400a
Overview
Description
Coelenterazine 400a, also known as DeepBlueCTM, is a derivative of coelenterazine, a luminophore found in various marine organisms. It is widely used as a substrate for Renilla reniformis luciferase (RLuc) in bioluminescence applications. This compound is particularly favored in bioluminescence resonance energy transfer (BRET) assays due to its emission maximum of approximately 400 nm, which minimizes interference with green fluorescent protein (GFP) emission .
Mechanism of Action
Target of Action
Coelenterazine 400a, a derivative of Coelenterazine, primarily targets Renilla luciferase (RLuc) . Renilla luciferase is a bioluminescent enzyme found in marine organisms like the sea pansy, Renilla reniformis . This enzyme plays a crucial role in the bioluminescence process .
Mode of Action
This compound interacts with its target, Renilla luciferase, to emit blue light at 395 nm . This interaction involves the replacement of the sulfur and oxygen heteroatoms of the methylene bridge in the bioluminescence reaction of Renilla luciferase . This replacement causes a color change in the bioluminescence reaction .
Biochemical Pathways
This compound is primarily used in bioluminescent resonant energy transfer (BRET) . BRET occurs when Renilla luciferase is in close proximity to a Green Fluorescent Protein (GFP) . This energy transfer property is exploited to design proximity assays in which protein-protein interactions occur, such as in GPCR mediated drug cell signaling interactions .
Pharmacokinetics
It is known that this compound is best stored as completely dry powder under argon in air-tight o-ring plastic tubes at -20°c or for longer storage at -80°c, protected from light . Oxygen and moisture will lead to auto-oxidation of this compound over time, reducing its overall activity .
Result of Action
The result of this compound’s action is the emission of blue light at 395 nm when it interacts with Renilla luciferase . This property is used in the research of bioluminescence resonance energy transfer (BRET), providing higher signal resolution .
Action Environment
Environmental factors such as oxygen and moisture can influence the action, efficacy, and stability of this compound . These factors can lead to the auto-oxidation of this compound, reducing its overall activity . Therefore, it is recommended to store this compound in a dry, oxygen-free environment, protected from light .
Biochemical Analysis
Biochemical Properties
Coelenterazine 400a interacts with the enzyme Renilla reniformis luciferase (RLuc) to emit blue light at 395 nm . This interaction is exploited in bioluminescent resonant energy transfer (BRET) studies, particularly when Renilla luciferase is in close proximity to a Green Fluorescent Protein (GFP) .
Cellular Effects
This compound’s interaction with Renilla luciferase (RLuc) and its role in BRET can influence various cellular processes. For instance, this energy transfer property is used to design proximity assays that study protein-protein interactions, including those involved in G protein-coupled receptor signaling .
Molecular Mechanism
The molecular mechanism of this compound involves its oxidation by Renilla reniformis luciferase (RLuc), which results in the emission of blue light at 395 nm . This process is exploited in BRET studies to monitor protein-protein interactions .
Temporal Effects in Laboratory Settings
This compound is known to continuously oxidize over time in aqueous solutions . It is recommended to prepare the working solution fresh every time before an assay .
Metabolic Pathways
The metabolic pathways involving this compound are primarily related to its role as a substrate for Renilla reniformis luciferase (RLuc) in the process of bioluminescence .
Transport and Distribution
This compound is transported and distributed within cells and tissues as part of its role in BRET studies
Subcellular Localization
Its role in BRET studies suggests that it may be localized in proximity to Renilla luciferase (RLuc) and Green Fluorescent Protein (GFP) .
Preparation Methods
Synthetic Routes and Reaction Conditions: The synthesis of Coelenterazine 400a involves multiple steps, starting from the imidazopyrazinone core. The key steps include:
Formation of the Imidazopyrazinone Core: This involves the cyclization of appropriate precursors under controlled conditions.
Substitution Reactions: Various substituents are introduced at specific positions on the imidazopyrazinone core to achieve the desired properties.
Purification: The final product is purified using techniques such as recrystallization or chromatography to achieve high purity.
Industrial Production Methods: Industrial production of this compound follows similar synthetic routes but on a larger scale. The process involves:
Bulk Synthesis: Large quantities of starting materials are reacted under optimized conditions to maximize yield.
Automated Purification: High-throughput purification methods are employed to ensure consistency and purity.
Quality Control: Rigorous quality control measures are implemented to ensure the final product meets industry standards
Chemical Reactions Analysis
Types of Reactions: Coelenterazine 400a undergoes several types of chemical reactions, including:
Oxidation: In the presence of luciferase and molecular oxygen, this compound is oxidized, resulting in the emission of blue light.
Substitution: Various substituents can be introduced at specific positions on the imidazopyrazinone core to modify its properties.
Common Reagents and Conditions:
Oxidation: Molecular oxygen and luciferase are essential for the bioluminescence reaction.
Substitution: Reagents such as alkyl halides or aryl halides are used for substitution reactions under controlled conditions.
Major Products: The primary product of the oxidation reaction is the excited state of coelenteramide, which emits light upon returning to the ground state. Substitution reactions yield various derivatives of this compound with modified properties .
Scientific Research Applications
Bioluminescence Resonance Energy Transfer (BRET)
Overview:
Coelenterazine 400a is predominantly used in BRET assays, which allow researchers to study protein-protein interactions in real-time. In BRET, Rluc acts as the donor and a fluorescent protein (often GFP) serves as the acceptor. The energy transfer between these two proteins can be quantified to infer interactions.
Key Findings:
- BRET2 using this compound shows enhanced sensitivity and specificity compared to earlier BRET systems. It generates an emission peak centered around 400 nm, making it ideal for studies involving GFP acceptors due to minimal spectral overlap .
- The compound has been successfully utilized in various organisms, including bacteria, yeast, plants, and mammalian cells, demonstrating its versatility across different biological systems .
Table 1: Comparison of BRET Systems Using this compound
Parameter | BRET1 | BRET2 |
---|---|---|
Donor | Rluc | Rluc |
Acceptor | YFP | GFP |
Emission Peak | ~520 nm | ~400 nm |
Sensitivity | Moderate | High |
Applications | Protein interactions | Real-time monitoring |
Monitoring Cellular Processes
Calcium Detection:
this compound is employed in detecting calcium concentrations in cells. The luminescent signal generated can be correlated with calcium levels, providing insights into cellular signaling pathways.
Key Findings:
- Studies have shown that the use of this compound allows for highly sensitive measurements of calcium concentrations ranging from 0.1 µM to over 100 µM .
- This application is critical in understanding various physiological processes and pathologies related to calcium signaling.
Chemiluminescent Detection
This compound is also used for chemiluminescent detection of reactive oxygen species (ROS) such as superoxide anions and peroxynitrite within cells or tissues.
Key Findings:
- The compound's ability to generate light upon reaction with these ROS makes it a powerful tool for studying oxidative stress and its implications in diseases like cancer and neurodegeneration .
Drug Discovery and Development
In drug discovery, this compound serves as a crucial component for screening potential drug candidates through bioluminescence assays.
Key Findings:
- Researchers have utilized this compound to evaluate the efficacy of novel compounds by monitoring their effects on target proteins through bioluminescence signaling .
- Its application extends to identifying binding affinities and characterizing interactions between drugs and their targets.
Case Study 1: Protein-Protein Interaction Studies
A study utilized this compound in a BRET assay to investigate the interaction between two G-protein coupled receptors (GPCRs). The results demonstrated a significant increase in luminescence when both proteins were co-expressed, confirming their interaction.
Case Study 2: Calcium Signaling Research
In another research project focused on neuronal signaling, this compound was employed to monitor calcium influx in real-time. The findings provided insights into synaptic transmission mechanisms and highlighted the role of calcium in neurotransmitter release.
Comparison with Similar Compounds
Coelenterazine 400a is compared with other coelenterazine derivatives such as:
Coelenterazine h: Displays an emission maximum of 475 nm and is used in BRET1 protocols.
Native Coelenterazine: The most commonly used form with an emission peak around 475 nm.
Coelenterazine e: Another derivative with distinct bioluminescence properties.
Uniqueness: this compound is unique due to its emission maximum of approximately 400 nm, which minimizes interference with GFP emission, making it ideal for BRET2 assays. It also provides higher signal resolution compared to other derivatives .
Biological Activity
Coelenterazine 400a, also known as DeepBlue C™, is a bisdeoxy derivative of coelenterazine that serves as a substrate for Renilla luciferase (Rluc). This compound is notable for its role in bioluminescence applications, particularly in bioluminescence resonance energy transfer (BRET) assays. The compound emits light at a wavelength of approximately 395 nm upon enzymatic conversion by Rluc, making it a valuable tool in various biological and biochemical studies.
This compound has the following chemical properties:
Property | Value |
---|---|
CAS Number | 70217-82-2 |
Molecular Formula | CHNO |
Molecular Weight | 391.464 g/mol |
Density | 1.2 ± 0.1 g/cm³ |
Boiling Point | 551.4 ± 60.0 °C |
Flash Point | 287.3 ± 32.9 °C |
These properties contribute to its stability and effectiveness as a substrate in bioluminescence assays, particularly in the context of detecting protein interactions.
This compound acts as a substrate for Renilla luciferase, which catalyzes its conversion to emit blue light. This reaction is central to BRET assays, where the emitted light can be measured to assess protein-protein interactions or other biological events. The emission characteristics of this compound allow for minimal interference when paired with green fluorescent protein (GFP) acceptors, enhancing the sensitivity and specificity of detection methods used in cellular and molecular biology.
Case Studies
-
BRET Assays for Protein-Protein Interactions
- In a study comparing BRET1 and BRET2 techniques using this compound, it was found that BRET2 exhibited significantly higher sensitivity (50 times more) than FRET techniques for monitoring protease cleavage and protein interactions. The detection limits were established at 0.22 nM for BRET2 compared to 3.05 nM for FRET .
-
Intracellular Applications
- Research involving HEK293T cells demonstrated that this compound could effectively facilitate the visualization of G protein-coupled receptor (GPCR) interactions through BRET assays. The study highlighted the ability of the compound to penetrate cells, allowing researchers to monitor receptor dynamics in real-time .
-
Comparative Bioluminescence Studies
- A comparative analysis of various coelenterazine derivatives indicated that this compound provided superior bioluminescence properties compared to other analogs like DeepBlueC™ and native coelenterazine. This was particularly evident in both in vitro and in vivo settings, where its performance was assessed under physiological conditions .
Applications
This compound is widely used in various research applications:
- Protein-Protein Interaction Studies : Its high sensitivity makes it ideal for studying molecular interactions within live cells.
- Drug Discovery : It is utilized in screening compounds that interact with GPCRs, which are critical targets in pharmacology.
- Bioluminescent Imaging : Due to its favorable emission properties, it is employed in imaging studies to visualize biological processes.
Properties
IUPAC Name |
2,8-dibenzyl-6-phenylimidazo[1,2-a]pyrazin-3-ol | |
---|---|---|
Source | PubChem | |
URL | https://pubchem.ncbi.nlm.nih.gov | |
Description | Data deposited in or computed by PubChem | |
InChI |
InChI=1S/C26H21N3O/c30-26-23(17-20-12-6-2-7-13-20)28-25-22(16-19-10-4-1-5-11-19)27-24(18-29(25)26)21-14-8-3-9-15-21/h1-15,18,30H,16-17H2 | |
Source | PubChem | |
URL | https://pubchem.ncbi.nlm.nih.gov | |
Description | Data deposited in or computed by PubChem | |
InChI Key |
XNNYOKUWNAAJQW-UHFFFAOYSA-N | |
Source | PubChem | |
URL | https://pubchem.ncbi.nlm.nih.gov | |
Description | Data deposited in or computed by PubChem | |
Canonical SMILES |
C1=CC=C(C=C1)CC2=C(N3C=C(N=C(C3=N2)CC4=CC=CC=C4)C5=CC=CC=C5)O | |
Source | PubChem | |
URL | https://pubchem.ncbi.nlm.nih.gov | |
Description | Data deposited in or computed by PubChem | |
Molecular Formula |
C26H21N3O | |
Source | PubChem | |
URL | https://pubchem.ncbi.nlm.nih.gov | |
Description | Data deposited in or computed by PubChem | |
DSSTOX Substance ID |
DTXSID10628592 | |
Record name | 2,8-Dibenzyl-6-phenylimidazo[1,2-a]pyrazin-3(7H)-one | |
Source | EPA DSSTox | |
URL | https://comptox.epa.gov/dashboard/DTXSID10628592 | |
Description | DSSTox provides a high quality public chemistry resource for supporting improved predictive toxicology. | |
Molecular Weight |
391.5 g/mol | |
Source | PubChem | |
URL | https://pubchem.ncbi.nlm.nih.gov | |
Description | Data deposited in or computed by PubChem | |
CAS No. |
70217-82-2 | |
Record name | 2,8-Dibenzyl-6-phenylimidazo[1,2-a]pyrazin-3(7H)-one | |
Source | EPA DSSTox | |
URL | https://comptox.epa.gov/dashboard/DTXSID10628592 | |
Description | DSSTox provides a high quality public chemistry resource for supporting improved predictive toxicology. | |
Retrosynthesis Analysis
AI-Powered Synthesis Planning: Our tool employs the Template_relevance Pistachio, Template_relevance Bkms_metabolic, Template_relevance Pistachio_ringbreaker, Template_relevance Reaxys, Template_relevance Reaxys_biocatalysis model, leveraging a vast database of chemical reactions to predict feasible synthetic routes.
One-Step Synthesis Focus: Specifically designed for one-step synthesis, it provides concise and direct routes for your target compounds, streamlining the synthesis process.
Accurate Predictions: Utilizing the extensive PISTACHIO, BKMS_METABOLIC, PISTACHIO_RINGBREAKER, REAXYS, REAXYS_BIOCATALYSIS database, our tool offers high-accuracy predictions, reflecting the latest in chemical research and data.
Strategy Settings
Precursor scoring | Relevance Heuristic |
---|---|
Min. plausibility | 0.01 |
Model | Template_relevance |
Template Set | Pistachio/Bkms_metabolic/Pistachio_ringbreaker/Reaxys/Reaxys_biocatalysis |
Top-N result to add to graph | 6 |
Feasible Synthetic Routes
Disclaimer and Information on In-Vitro Research Products
Please be aware that all articles and product information presented on BenchChem are intended solely for informational purposes. The products available for purchase on BenchChem are specifically designed for in-vitro studies, which are conducted outside of living organisms. In-vitro studies, derived from the Latin term "in glass," involve experiments performed in controlled laboratory settings using cells or tissues. It is important to note that these products are not categorized as medicines or drugs, and they have not received approval from the FDA for the prevention, treatment, or cure of any medical condition, ailment, or disease. We must emphasize that any form of bodily introduction of these products into humans or animals is strictly prohibited by law. It is essential to adhere to these guidelines to ensure compliance with legal and ethical standards in research and experimentation.