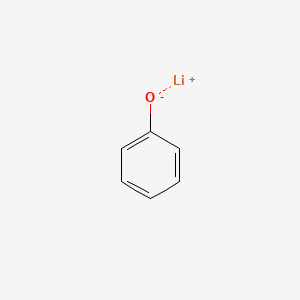
Lithium phenoxide
Overview
Description
Lithium phenoxide, also known as phenol lithium salt, is an organolithium compound with the chemical formula C6H5OLi. It is commonly used in organic synthesis and serves as a reagent in various chemical reactions. The compound is typically found as a solution in tetrahydrofuran (THF) and is known for its reactivity and utility in forming carbon-carbon bonds.
Preparation Methods
Synthetic Routes and Reaction Conditions: Lithium phenoxide can be prepared by reacting phenol with lithium metal in an aprotic solvent such as toluene. The reaction is typically carried out under a nitrogen atmosphere to prevent oxidation. The mixture is heated under reflux with stirring for an extended period, usually around 36 hours. The resulting this compound is then isolated by removing the solvent under reduced pressure and washing the residue with hexane .
Industrial Production Methods: In industrial settings, this compound is produced using similar methods but on a larger scale. The process involves the use of high-purity phenol and lithium metal, and the reaction is conducted in large reactors equipped with efficient stirring and temperature control systems. The product is then purified and concentrated to the desired specifications.
Types of Reactions:
Oxidation: this compound can undergo oxidation to form quinones. This reaction typically requires strong oxidizing agents such as potassium permanganate or chromium trioxide.
Reduction: The compound can be reduced to form phenol using reducing agents like lithium aluminum hydride.
Substitution: this compound is highly reactive towards electrophilic aromatic substitution reactions. It can react with various electrophiles to form substituted phenols.
Common Reagents and Conditions:
Oxidation: Potassium permanganate, chromium trioxide.
Reduction: Lithium aluminum hydride.
Substitution: Electrophiles such as alkyl halides, acyl chlorides.
Major Products Formed:
Oxidation: Quinones.
Reduction: Phenol.
Substitution: Substituted phenols.
Scientific Research Applications
Chemical Properties and Mechanism of Action
Lithium phenoxide is typically utilized as a reagent in organic synthesis, particularly in the formation of carbon-carbon bonds. Its unique properties stem from the small ionic radius of lithium, which allows for effective interactions with electrophiles, making it more reactive than sodium or potassium phenoxide counterparts.
Mechanism of Action:
- Target Action: Competes for sites specific to other cations such as sodium and magnesium.
- Biochemical Pathways: Influences neurotransmitter pathways, particularly dopamine, which can have implications in neuroprotection and mood stabilization.
Scientific Research Applications
This compound finds applications in several domains:
-
Organic Synthesis:
- Acts as a catalyst in various reactions, enabling the formation of complex organic molecules.
- Used in nucleophilic aromatic substitution reactions due to its high reactivity.
-
Pharmaceuticals:
- Integral in synthesizing biologically active compounds, including drugs used for treating mood disorders and neurodegenerative diseases.
- Electrochemical Applications:
-
Materials Science:
- Employed in producing advanced materials such as polymers and resins, contributing to the development of high-performance materials used in various industries.
Case Studies
Case Study 1: Neuroprotective Properties
A study highlighted the neuroprotective effects of lithium compounds, where this compound showed potential in reducing apoptosis in neuronal cells. This suggests its possible role in therapies for neurodegenerative conditions.
Case Study 2: Electrochemical Performance
Research comparing this compound with other proton shuttles in electrochemical cells revealed that it led to more stable electrode deposits and improved efficiency during ammonia synthesis. The findings underscore its importance in advancing electrochemical technologies .
Mechanism of Action
The mechanism of action of lithium phenoxide involves its ability to act as a nucleophile in various chemical reactions. The compound can donate its lone pair of electrons on the oxygen atom to form bonds with electrophiles. This nucleophilic behavior is crucial in many organic synthesis reactions, including the formation of carbon-carbon bonds and the substitution of aromatic rings.
Comparison with Similar Compounds
Sodium phenoxide: Similar to lithium phenoxide but uses sodium instead of lithium. It is less reactive due to the larger ionic radius of sodium.
Potassium phenoxide: Another similar compound with potassium. It is even less reactive than sodium phenoxide due to the larger size of potassium ions.
Uniqueness of this compound: this compound is unique due to its high reactivity and ability to form strong bonds with electrophiles. The small ionic radius of lithium allows for closer interaction with the aromatic ring, making it more effective in nucleophilic aromatic substitution reactions compared to its sodium and potassium counterparts .
Biological Activity
Lithium phenoxide, a compound formed from lithium and phenol, has garnered attention for its potential biological activities, particularly in the fields of pharmacology and materials science. This article explores the biological activity of this compound, focusing on its mechanisms, applications, and relevant research findings.
This compound (C₆H₅LiO) is an organometallic compound characterized by the presence of a lithium ion bonded to a phenoxide group. Its structure allows it to participate in various chemical reactions, making it a versatile compound in both organic synthesis and biological applications. The compound is notable for its high reactivity and ability to act as a catalyst in several reactions.
Mechanisms of Biological Activity
1. Neuroprotective Effects:
Lithium compounds have been extensively studied for their neuroprotective properties, particularly in the treatment of mood disorders such as bipolar disorder. This compound may exhibit similar properties due to its lithium content, which has been associated with mood stabilization and neuroprotection through mechanisms such as:
- Inhibition of Glycogen Synthase Kinase 3 (GSK-3): Lithium is known to inhibit GSK-3, an enzyme involved in various cellular processes including neuronal survival.
- Modulation of Neurotransmitter Systems: Lithium influences neurotransmitter levels, particularly serotonin and norepinephrine, which are critical in mood regulation.
2. Catalytic Activity:
this compound has been shown to function as a catalyst in various organic reactions. For instance, it catalyzes the addition of propylene oxide to phenol, facilitating the formation of valuable chemical intermediates. The reaction mechanism involves the formation of a transition state that lowers the activation energy required for the reaction to proceed .
Research Findings
Recent studies have highlighted several aspects of this compound's biological activity:
- Ammonia Synthesis: this compound has been utilized in lithium-mediated nitrogen reduction reactions (Li-NRR), demonstrating effectiveness in synthesizing ammonia under ambient conditions. This process showcases its potential application in sustainable chemistry .
- Proton Shuttle Mechanism: Research indicates that this compound can act as a proton shuttle during electrochemical processes, enhancing Faradaic efficiency in ammonia production compared to traditional methods using phenol alone . This property could be pivotal for developing more efficient electrochemical systems.
Case Studies
Case Study 1: Neuroprotective Properties
A study examining the effects of lithium on neuronal health found that lithium compounds can significantly reduce apoptosis (programmed cell death) in neuronal cells. The study suggested that this compound might share these protective effects due to its structural similarities with other lithium compounds .
Case Study 2: Electrochemical Applications
In a comparative analysis of this compound and other proton shuttles in electrochemical cells, researchers found that this compound led to more stable electrode deposits and improved overall efficiency during ammonia synthesis. This study emphasized the importance of this compound in advancing electrochemical technologies .
Data Tables
Property | This compound | Phenol |
---|---|---|
Molecular Weight | 106.11 g/mol | 94.11 g/mol |
Solubility | Soluble in organic solvents | Soluble in water |
Catalytic Activity | High | Moderate |
Neuroprotective Potential | Yes | Limited |
Chemical Reactions Analysis
Kolbe-Schmitt Reaction
The Kolbe-Schmitt reaction involves the carboxylation of lithium phenoxide with carbon dioxide, leading to the formation of salicylic acid. The mechanism includes several key steps:
-
Formation of Intermediates: The reaction proceeds through multiple transition states and intermediates, characterized by distinct geometrical configurations .
-
Activation Energy: Studies have shown that the apparent activation energy for this reaction is approximately 10.6 kcal/mol, which is lower than that observed for sodium phenoxide .
Addition of Propylene Oxide
This compound acts as a catalyst in the addition of propylene oxide to phenol. This reaction has been studied under various temperatures and molar ratios of reactants:
-
Kinetic Studies: The reaction exhibits a non-first-order behavior concerning this compound concentration, suggesting complex interactions among reactants .
-
Reaction Order: The order of reaction tends to be approximately with respect to this compound concentration, indicating a possible equilibrium between monomeric and oligomeric forms of the catalyst .
-
Research Findings
Recent studies have highlighted several key findings regarding this compound:
-
Neuroprotective Properties: Research indicates that lithium compounds, including this compound, may offer neuroprotective effects by modulating neurotransmitter pathways and reducing neuronal apoptosis.
-
Electrochemical Applications: this compound has shown promise in electrochemical applications, particularly in improving the efficiency of ammonia synthesis processes due to its unique properties compared to other alkali metal phenoxides .
-
Comparison with Related Compounds
The reactivity of this compound can be contrasted with sodium and potassium phenoxides:
Compound | Reactivity Level | Comments |
---|---|---|
This compound | High | Small ionic radius enhances nucleophilicity |
Sodium Phenoxide | Moderate | Larger ionic radius reduces reactivity |
Potassium Phenoxide | Low | Least reactive due to even larger size |
This compound is a versatile compound with significant implications in organic synthesis and biochemical applications. Its unique reactivity profile allows it to participate in various chemical reactions, making it valuable in both laboratory research and industrial applications. Further studies are warranted to explore its full potential across different fields, including materials science and pharmaceuticals.
Properties
CAS No. |
555-24-8 |
---|---|
Molecular Formula |
C6H6LiO |
Molecular Weight |
101.1 g/mol |
IUPAC Name |
lithium;phenoxide |
InChI |
InChI=1S/C6H6O.Li/c7-6-4-2-1-3-5-6;/h1-5,7H; |
InChI Key |
WAVGXIXQMBSEMK-UHFFFAOYSA-N |
SMILES |
[Li+].C1=CC=C(C=C1)[O-] |
Canonical SMILES |
[Li].C1=CC=C(C=C1)O |
Key on ui other cas no. |
555-24-8 |
Pictograms |
Flammable; Corrosive |
Origin of Product |
United States |
Retrosynthesis Analysis
AI-Powered Synthesis Planning: Our tool employs the Template_relevance Pistachio, Template_relevance Bkms_metabolic, Template_relevance Pistachio_ringbreaker, Template_relevance Reaxys, Template_relevance Reaxys_biocatalysis model, leveraging a vast database of chemical reactions to predict feasible synthetic routes.
One-Step Synthesis Focus: Specifically designed for one-step synthesis, it provides concise and direct routes for your target compounds, streamlining the synthesis process.
Accurate Predictions: Utilizing the extensive PISTACHIO, BKMS_METABOLIC, PISTACHIO_RINGBREAKER, REAXYS, REAXYS_BIOCATALYSIS database, our tool offers high-accuracy predictions, reflecting the latest in chemical research and data.
Strategy Settings
Precursor scoring | Relevance Heuristic |
---|---|
Min. plausibility | 0.01 |
Model | Template_relevance |
Template Set | Pistachio/Bkms_metabolic/Pistachio_ringbreaker/Reaxys/Reaxys_biocatalysis |
Top-N result to add to graph | 6 |
Feasible Synthetic Routes
Disclaimer and Information on In-Vitro Research Products
Please be aware that all articles and product information presented on BenchChem are intended solely for informational purposes. The products available for purchase on BenchChem are specifically designed for in-vitro studies, which are conducted outside of living organisms. In-vitro studies, derived from the Latin term "in glass," involve experiments performed in controlled laboratory settings using cells or tissues. It is important to note that these products are not categorized as medicines or drugs, and they have not received approval from the FDA for the prevention, treatment, or cure of any medical condition, ailment, or disease. We must emphasize that any form of bodily introduction of these products into humans or animals is strictly prohibited by law. It is essential to adhere to these guidelines to ensure compliance with legal and ethical standards in research and experimentation.