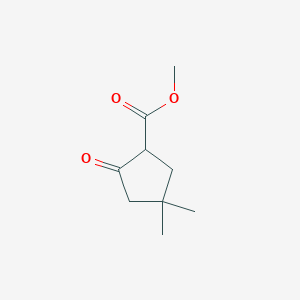
Methyl 4,4-dimethyl-2-oxocyclopentanecarboxylate
Overview
Description
Methyl 4,4-dimethyl-2-oxocyclopentanecarboxylate is an organic compound with the molecular formula C10H16O3. It is a derivative of cyclopentanecarboxylate and is characterized by the presence of a methyl ester group and a ketone functional group on a cyclopentane ring. This compound is used in various chemical reactions and has applications in scientific research and industry.
Preparation Methods
Synthetic Routes and Reaction Conditions
Methyl 4,4-dimethyl-2-oxocyclopentanecarboxylate can be synthesized through several synthetic routes. One common method involves the reaction of 4,4-dimethyl-2-oxocyclopentanone with methanol in the presence of an acid catalyst. The reaction typically proceeds under reflux conditions, and the product is purified through distillation or recrystallization.
Industrial Production Methods
In industrial settings, the production of this compound may involve large-scale batch or continuous processes. The reaction conditions are optimized to maximize yield and purity, and advanced purification techniques such as chromatography may be employed to ensure the quality of the final product.
Chemical Reactions Analysis
Types of Reactions
Methyl 4,4-dimethyl-2-oxocyclopentanecarboxylate undergoes various chemical reactions, including:
Oxidation: The compound can be oxidized to form corresponding carboxylic acids or other oxidized derivatives.
Reduction: Reduction reactions can convert the ketone group to an alcohol, resulting in the formation of methyl 4,4-dimethyl-2-hydroxycyclopentanecarboxylate.
Substitution: The ester group can undergo nucleophilic substitution reactions, leading to the formation of different ester derivatives.
Common Reagents and Conditions
Oxidation: Common oxidizing agents include potassium permanganate (KMnO4) and chromium trioxide (CrO3).
Reduction: Reducing agents such as sodium borohydride (NaBH4) or lithium aluminum hydride (LiAlH4) are typically used.
Substitution: Nucleophiles such as alcohols or amines can be used in substitution reactions, often in the presence of a base or acid catalyst.
Major Products Formed
Oxidation: Carboxylic acids or ketone derivatives.
Reduction: Alcohol derivatives.
Substitution: Various ester or amide derivatives.
Scientific Research Applications
Methyl 4,4-dimethyl-2-oxocyclopentanecarboxylate has several applications in scientific research:
Chemistry: It is used as an intermediate in the synthesis of complex organic molecules and as a reagent in various chemical reactions.
Biology: The compound may be used in the study of enzyme-catalyzed reactions and metabolic pathways.
Medicine: Research into potential pharmaceutical applications, including drug development and the study of biological activity.
Industry: Utilized in the production of fine chemicals, agrochemicals, and other industrial products.
Mechanism of Action
The mechanism of action of methyl 4,4-dimethyl-2-oxocyclopentanecarboxylate depends on the specific reaction or application. In general, the compound interacts with molecular targets through its functional groups, such as the ester and ketone groups. These interactions can involve nucleophilic attack, electrophilic addition, or other chemical processes, leading to the formation of new chemical bonds and products.
Comparison with Similar Compounds
Similar Compounds
- Methyl 2-oxo-4,4-dimethylcyclopentanecarboxylate
- 4,4-Dimethyl-2-oxocyclopentanecarboxylic acid methyl ester
Uniqueness
Methyl 4,4-dimethyl-2-oxocyclopentanecarboxylate is unique due to its specific structural features, such as the presence of both a ketone and an ester group on a cyclopentane ring. This combination of functional groups allows it to participate in a wide range of chemical reactions and makes it a valuable intermediate in organic synthesis.
Properties
IUPAC Name |
methyl 4,4-dimethyl-2-oxocyclopentane-1-carboxylate | |
---|---|---|
Source | PubChem | |
URL | https://pubchem.ncbi.nlm.nih.gov | |
Description | Data deposited in or computed by PubChem | |
InChI |
InChI=1S/C9H14O3/c1-9(2)4-6(7(10)5-9)8(11)12-3/h6H,4-5H2,1-3H3 | |
Source | PubChem | |
URL | https://pubchem.ncbi.nlm.nih.gov | |
Description | Data deposited in or computed by PubChem | |
InChI Key |
XWEUDAXDKUDFMQ-UHFFFAOYSA-N | |
Source | PubChem | |
URL | https://pubchem.ncbi.nlm.nih.gov | |
Description | Data deposited in or computed by PubChem | |
Canonical SMILES |
CC1(CC(C(=O)C1)C(=O)OC)C | |
Source | PubChem | |
URL | https://pubchem.ncbi.nlm.nih.gov | |
Description | Data deposited in or computed by PubChem | |
Molecular Formula |
C9H14O3 | |
Source | PubChem | |
URL | https://pubchem.ncbi.nlm.nih.gov | |
Description | Data deposited in or computed by PubChem | |
DSSTOX Substance ID |
DTXSID50472778 | |
Record name | METHYL 4,4-DIMETHYL-2-OXOCYCLOPENTANECARBOXYLATE | |
Source | EPA DSSTox | |
URL | https://comptox.epa.gov/dashboard/DTXSID50472778 | |
Description | DSSTox provides a high quality public chemistry resource for supporting improved predictive toxicology. | |
Molecular Weight |
170.21 g/mol | |
Source | PubChem | |
URL | https://pubchem.ncbi.nlm.nih.gov | |
Description | Data deposited in or computed by PubChem | |
CAS No. |
60585-44-6 | |
Record name | METHYL 4,4-DIMETHYL-2-OXOCYCLOPENTANECARBOXYLATE | |
Source | EPA DSSTox | |
URL | https://comptox.epa.gov/dashboard/DTXSID50472778 | |
Description | DSSTox provides a high quality public chemistry resource for supporting improved predictive toxicology. | |
Record name | methyl 4,4-dimethyl-2-oxocyclopentane-1-carboxylate | |
Source | European Chemicals Agency (ECHA) | |
URL | https://echa.europa.eu/information-on-chemicals | |
Description | The European Chemicals Agency (ECHA) is an agency of the European Union which is the driving force among regulatory authorities in implementing the EU's groundbreaking chemicals legislation for the benefit of human health and the environment as well as for innovation and competitiveness. | |
Explanation | Use of the information, documents and data from the ECHA website is subject to the terms and conditions of this Legal Notice, and subject to other binding limitations provided for under applicable law, the information, documents and data made available on the ECHA website may be reproduced, distributed and/or used, totally or in part, for non-commercial purposes provided that ECHA is acknowledged as the source: "Source: European Chemicals Agency, http://echa.europa.eu/". Such acknowledgement must be included in each copy of the material. ECHA permits and encourages organisations and individuals to create links to the ECHA website under the following cumulative conditions: Links can only be made to webpages that provide a link to the Legal Notice page. | |
Retrosynthesis Analysis
AI-Powered Synthesis Planning: Our tool employs the Template_relevance Pistachio, Template_relevance Bkms_metabolic, Template_relevance Pistachio_ringbreaker, Template_relevance Reaxys, Template_relevance Reaxys_biocatalysis model, leveraging a vast database of chemical reactions to predict feasible synthetic routes.
One-Step Synthesis Focus: Specifically designed for one-step synthesis, it provides concise and direct routes for your target compounds, streamlining the synthesis process.
Accurate Predictions: Utilizing the extensive PISTACHIO, BKMS_METABOLIC, PISTACHIO_RINGBREAKER, REAXYS, REAXYS_BIOCATALYSIS database, our tool offers high-accuracy predictions, reflecting the latest in chemical research and data.
Strategy Settings
Precursor scoring | Relevance Heuristic |
---|---|
Min. plausibility | 0.01 |
Model | Template_relevance |
Template Set | Pistachio/Bkms_metabolic/Pistachio_ringbreaker/Reaxys/Reaxys_biocatalysis |
Top-N result to add to graph | 6 |
Feasible Synthetic Routes
Disclaimer and Information on In-Vitro Research Products
Please be aware that all articles and product information presented on BenchChem are intended solely for informational purposes. The products available for purchase on BenchChem are specifically designed for in-vitro studies, which are conducted outside of living organisms. In-vitro studies, derived from the Latin term "in glass," involve experiments performed in controlled laboratory settings using cells or tissues. It is important to note that these products are not categorized as medicines or drugs, and they have not received approval from the FDA for the prevention, treatment, or cure of any medical condition, ailment, or disease. We must emphasize that any form of bodily introduction of these products into humans or animals is strictly prohibited by law. It is essential to adhere to these guidelines to ensure compliance with legal and ethical standards in research and experimentation.