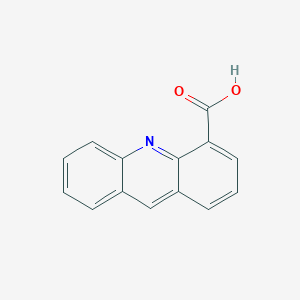
Acridine-4-carboxylic Acid
Overview
Description
Acridine-4-carboxylic acid is a derivative of acridine, a nitrogen-containing heterocyclic compound. Acridine derivatives are known for their broad range of pharmaceutical properties, including anti-inflammatory, anticancer, antimicrobial, antitubercular, antiparasitic, antimalarial, antiviral, and fungicidal activities
Mechanism of Action
Target of Action
The primary target of Acridine-4-carboxylic Acid is DNA . Acridine derivatives, including this compound, are known to intercalate into double-stranded DNA . This interaction is facilitated by the planar structure of acridine, which allows it to sandwich between the base pairs of the double helix .
Mode of Action
This compound interacts with its target, DNA, through a process called intercalation . This process involves the insertion of the planar acridine molecule between the base pairs of the DNA double helix . This intercalation is driven by charge transfer and π-stacking interactions . The result of this interaction is the unwinding of the helical structure of DNA .
Biochemical Pathways
The intercalation of this compound into DNA affects various biological processes involving DNA and related enzymes . This interaction can lead to the inhibition of DNA replication and transcription, thereby affecting the normal functioning of cells . The exact biochemical pathways affected by this compound can vary depending on the specific cellular context.
Pharmacokinetics
The ability of acridine derivatives to intercalate into dna suggests that they can penetrate cellular membranes and reach their target sites .
Result of Action
The intercalation of this compound into DNA can lead to the inhibition of DNA replication and transcription . This can result in the disruption of normal cellular processes, potentially leading to cell death . This property has led to the investigation of acridine derivatives as potential therapeutic agents for various disorders, including cancer .
Action Environment
The action of this compound can be influenced by various environmental factors. For instance, the presence of other bioactive substances, such as DNA repair protein inhibitors, can enhance the anticancer effects of acridine medications . Furthermore, the structural features of acridine photocatalysts can influence the efficiency of decarboxylative transformations . .
Biochemical Analysis
Biochemical Properties
Acridine-4-carboxylic Acid plays a crucial role in biochemical reactions, particularly in its interaction with heme. It is a heme-interacting acridone derivative that prevents free heme-mediated protein oxidation and degradation . This compound inhibits protein carbonyl formation with an IC50 of 43 μM . This interaction is significant as it helps in protecting proteins from oxidative damage, thereby maintaining cellular integrity.
Cellular Effects
This compound has been shown to influence various cellular processes. It exhibits cytotoxic activity against cancer cell lines, including MDA-MB-231, SK-BR-3, and MCF-7 . The compound intercalates into DNA, disrupting the helical structure and affecting DNA replication and transcription . This disruption can lead to changes in gene expression and cellular metabolism, ultimately influencing cell function and viability.
Molecular Mechanism
The molecular mechanism of this compound involves its ability to intercalate into DNA and inhibit topoisomerase enzymes . By intercalating into the DNA, the compound disrupts the normal function of topoisomerases, leading to the inhibition of DNA replication and transcription . Additionally, this compound can form complexes with heme, preventing heme-mediated oxidative damage to proteins .
Temporal Effects in Laboratory Settings
In laboratory settings, the effects of this compound have been observed to change over time. The compound is stable under various storage conditions, including -20°C for up to 3 years and -80°C for up to 6 months . Long-term studies have shown that this compound can maintain its protective effects against protein oxidation over extended periods . Its stability and efficacy may vary depending on the experimental conditions and the presence of other reactive species.
Dosage Effects in Animal Models
The effects of this compound vary with different dosages in animal models. Studies have shown that the compound exhibits anti-tumor activity at doses of 12.5, 25, or 50 mg/kg in the Ehrlich ascites carcinoma model . At higher doses, this compound may exhibit toxic or adverse effects, highlighting the importance of dosage optimization in therapeutic applications .
Metabolic Pathways
This compound is involved in various metabolic pathways, particularly those related to its interaction with heme and DNA. The compound can be metabolized by enzymes such as mono- or dioxygenases, which facilitate its degradation and transformation . These metabolic pathways are crucial for the compound’s bioavailability and efficacy in therapeutic applications.
Transport and Distribution
Within cells and tissues, this compound is transported and distributed through interactions with transporters and binding proteins. The compound’s ability to intercalate into DNA and form complexes with heme influences its localization and accumulation in specific cellular compartments . These interactions are essential for its biological activity and therapeutic potential.
Subcellular Localization
This compound exhibits specific subcellular localization, primarily within the nucleus due to its DNA-intercalating properties . The compound’s localization is influenced by targeting signals and post-translational modifications that direct it to specific compartments or organelles. This subcellular localization is critical for its function in regulating gene expression and protecting proteins from oxidative damage.
Preparation Methods
Synthetic Routes and Reaction Conditions: Acridine-4-carboxylic acid can be synthesized through several methods. One common approach involves the condensation of diphenylamine with carboxylic acids in the presence of zinc chloride. When formic acid is used as the carboxylic acid, the reaction yields the parent acridine . For this compound, specific carboxylic acids are used to introduce the carboxyl group at the 4-position of the acridine ring.
Industrial Production Methods: Industrial production of this compound typically involves large-scale synthesis using optimized reaction conditions to ensure high yield and purity. The use of advanced catalytic processes and continuous flow reactors can enhance the efficiency of the production process.
Chemical Reactions Analysis
Types of Reactions: Acridine-4-carboxylic acid undergoes various chemical reactions, including oxidation, reduction, and substitution reactions.
Common Reagents and Conditions:
Reduction: Reduction of this compound can be achieved using reducing agents like lithium aluminum hydride or sodium borohydride.
Substitution: Substitution reactions can occur at different positions on the acridine ring, depending on the reagents and conditions used. For example, halogenation can be achieved using halogens like chlorine or bromine in the presence of a catalyst.
Major Products: The major products formed from these reactions include acridinic acid, acridone, and various substituted acridine derivatives.
Scientific Research Applications
Acridine-4-carboxylic acid has a wide range of scientific research applications:
Comparison with Similar Compounds
Acridine-4-carboxylic acid can be compared with other acridine derivatives such as:
N-(2-(dimethylamino)ethyl)acridine-4-carboxamide (DACA): Known for its DNA intercalating properties and anticancer activity.
Triazoloacridone (C-1305): Exhibits potent antitumor activity and is being studied for its potential clinical applications.
Amsacrine (m-AMSA): A clinically used anticancer drug that intercalates DNA and inhibits topoisomerase II.
The uniqueness of this compound lies in its specific substitution pattern, which imparts distinct chemical and biological properties compared to other acridine derivatives.
Properties
IUPAC Name |
acridine-4-carboxylic acid | |
---|---|---|
Source | PubChem | |
URL | https://pubchem.ncbi.nlm.nih.gov | |
Description | Data deposited in or computed by PubChem | |
InChI |
InChI=1S/C14H9NO2/c16-14(17)11-6-3-5-10-8-9-4-1-2-7-12(9)15-13(10)11/h1-8H,(H,16,17) | |
Source | PubChem | |
URL | https://pubchem.ncbi.nlm.nih.gov | |
Description | Data deposited in or computed by PubChem | |
InChI Key |
NBIUCDPMOWCGIG-UHFFFAOYSA-N | |
Source | PubChem | |
URL | https://pubchem.ncbi.nlm.nih.gov | |
Description | Data deposited in or computed by PubChem | |
Canonical SMILES |
C1=CC=C2C(=C1)C=C3C=CC=C(C3=N2)C(=O)O | |
Source | PubChem | |
URL | https://pubchem.ncbi.nlm.nih.gov | |
Description | Data deposited in or computed by PubChem | |
Molecular Formula |
C14H9NO2 | |
Source | PubChem | |
URL | https://pubchem.ncbi.nlm.nih.gov | |
Description | Data deposited in or computed by PubChem | |
DSSTOX Substance ID |
DTXSID80456642 | |
Record name | Acridine-4-carboxylic Acid | |
Source | EPA DSSTox | |
URL | https://comptox.epa.gov/dashboard/DTXSID80456642 | |
Description | DSSTox provides a high quality public chemistry resource for supporting improved predictive toxicology. | |
Molecular Weight |
223.23 g/mol | |
Source | PubChem | |
URL | https://pubchem.ncbi.nlm.nih.gov | |
Description | Data deposited in or computed by PubChem | |
CAS No. |
31327-97-6 | |
Record name | Acridine-4-carboxylic Acid | |
Source | EPA DSSTox | |
URL | https://comptox.epa.gov/dashboard/DTXSID80456642 | |
Description | DSSTox provides a high quality public chemistry resource for supporting improved predictive toxicology. | |
Synthesis routes and methods
Procedure details
Retrosynthesis Analysis
AI-Powered Synthesis Planning: Our tool employs the Template_relevance Pistachio, Template_relevance Bkms_metabolic, Template_relevance Pistachio_ringbreaker, Template_relevance Reaxys, Template_relevance Reaxys_biocatalysis model, leveraging a vast database of chemical reactions to predict feasible synthetic routes.
One-Step Synthesis Focus: Specifically designed for one-step synthesis, it provides concise and direct routes for your target compounds, streamlining the synthesis process.
Accurate Predictions: Utilizing the extensive PISTACHIO, BKMS_METABOLIC, PISTACHIO_RINGBREAKER, REAXYS, REAXYS_BIOCATALYSIS database, our tool offers high-accuracy predictions, reflecting the latest in chemical research and data.
Strategy Settings
Precursor scoring | Relevance Heuristic |
---|---|
Min. plausibility | 0.01 |
Model | Template_relevance |
Template Set | Pistachio/Bkms_metabolic/Pistachio_ringbreaker/Reaxys/Reaxys_biocatalysis |
Top-N result to add to graph | 6 |
Feasible Synthetic Routes
Disclaimer and Information on In-Vitro Research Products
Please be aware that all articles and product information presented on BenchChem are intended solely for informational purposes. The products available for purchase on BenchChem are specifically designed for in-vitro studies, which are conducted outside of living organisms. In-vitro studies, derived from the Latin term "in glass," involve experiments performed in controlled laboratory settings using cells or tissues. It is important to note that these products are not categorized as medicines or drugs, and they have not received approval from the FDA for the prevention, treatment, or cure of any medical condition, ailment, or disease. We must emphasize that any form of bodily introduction of these products into humans or animals is strictly prohibited by law. It is essential to adhere to these guidelines to ensure compliance with legal and ethical standards in research and experimentation.