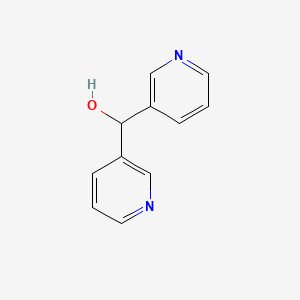
Di(pyridin-3-yl)methanol
Overview
Description
Di(pyridin-3-yl)methanol is an organic compound with the molecular formula C11H10N2O. It is characterized by the presence of two pyridine rings attached to a central methanol group. This compound is known for its stability and solubility in common organic solvents such as ethanol, methanol, and dimethylformamide. It is used in various chemical reactions and has applications in scientific research.
Biochemical Analysis
Biochemical Properties
Di(pyridin-3-yl)methanol plays a significant role in biochemical reactions, particularly in its interactions with enzymes and proteins. It has been observed to interact with various enzymes, including those involved in oxidation-reduction reactions. The compound’s structure allows it to form hydrogen bonds and π-π interactions with amino acid residues in enzyme active sites, thereby influencing enzyme activity. For instance, this compound can act as a ligand for metal ions in metalloproteins, affecting their catalytic functions .
Cellular Effects
This compound has notable effects on cellular processes. It influences cell signaling pathways, gene expression, and cellular metabolism. Studies have shown that this compound can modulate the activity of key signaling molecules, such as kinases and phosphatases, leading to altered phosphorylation states of target proteins. This modulation can impact various cellular functions, including cell growth, differentiation, and apoptosis . Additionally, this compound has been found to affect the expression of genes involved in metabolic pathways, thereby influencing cellular energy production and biosynthesis[3][3].
Molecular Mechanism
The molecular mechanism of this compound involves its binding interactions with biomolecules. The compound can bind to enzyme active sites, either inhibiting or activating their catalytic activities. For example, this compound can inhibit the activity of certain oxidoreductases by binding to their active sites and preventing substrate access . Furthermore, the compound can induce changes in gene expression by interacting with transcription factors and other regulatory proteins, thereby influencing the transcriptional machinery .
Temporal Effects in Laboratory Settings
In laboratory settings, the effects of this compound can change over time. The compound’s stability and degradation are critical factors that influence its long-term effects on cellular function. This compound is relatively stable under standard laboratory conditions, but it can undergo degradation when exposed to light or extreme pH conditions . Long-term studies have shown that prolonged exposure to this compound can lead to cumulative effects on cellular processes, such as sustained changes in gene expression and metabolic flux .
Dosage Effects in Animal Models
The effects of this compound vary with different dosages in animal models. At low doses, the compound can enhance certain biochemical pathways without causing significant toxicity. At higher doses, this compound can exhibit toxic effects, including oxidative stress and cellular damage . Threshold effects have been observed, where a specific dosage range is required to achieve the desired biochemical effects without inducing adverse reactions .
Metabolic Pathways
This compound is involved in various metabolic pathways, interacting with enzymes and cofactors that facilitate its conversion and utilization. The compound can be metabolized through oxidation-reduction reactions, leading to the formation of intermediate metabolites . These metabolic pathways can influence the overall metabolic flux and levels of key metabolites within cells .
Transport and Distribution
Within cells and tissues, this compound is transported and distributed through interactions with specific transporters and binding proteins. The compound can be taken up by cells via passive diffusion or active transport mechanisms . Once inside the cell, this compound can bind to intracellular proteins, influencing its localization and accumulation in specific cellular compartments .
Subcellular Localization
The subcellular localization of this compound is influenced by targeting signals and post-translational modifications. The compound can be directed to specific organelles, such as the mitochondria or nucleus, where it exerts its biochemical effects . Post-translational modifications, such as phosphorylation or acetylation, can further regulate the activity and function of this compound within these subcellular compartments .
Preparation Methods
Synthetic Routes and Reaction Conditions
Di(pyridin-3-yl)methanol can be synthesized through the reaction of pyridine with benzyl bromide in the presence of a base catalyst. The process involves adding benzyl bromide to an organic solvent containing pyridine, followed by the addition of a base catalyst. The reaction mixture is then stirred for a specific period, and the product is purified through a series of steps to obtain the desired compound .
Industrial Production Methods
In industrial settings, the production of this compound typically involves large-scale reactions using similar synthetic routes. The process is optimized for higher yields and purity, often employing advanced purification techniques such as recrystallization and chromatography to ensure the quality of the final product.
Chemical Reactions Analysis
Types of Reactions
Di(pyridin-3-yl)methanol undergoes various chemical reactions, including:
Reduction: It can be reduced under specific conditions to yield different derivatives.
Substitution: The methanol group can be substituted with other functional groups through nucleophilic substitution reactions.
Common Reagents and Conditions
Oxidation: Copper catalysts and water are commonly used for the oxidation of this compound.
Reduction: Reducing agents such as lithium aluminum hydride can be used.
Substitution: Nucleophiles such as halides and amines are employed in substitution reactions.
Major Products Formed
Oxidation: Pyridin-3-yl-methanone.
Reduction: Various reduced derivatives depending on the reducing agent used.
Substitution: Substituted pyridin-3-yl derivatives.
Scientific Research Applications
Di(pyridin-3-yl)methanol has a wide range of applications in scientific research:
Mechanism of Action
The mechanism by which di(pyridin-3-yl)methanol exerts its effects involves its interaction with specific molecular targets and pathways. In biological systems, it can bind to enzymes and receptors, modulating their activity. The compound’s structure allows it to participate in various biochemical reactions, influencing cellular processes and pathways .
Comparison with Similar Compounds
Di(pyridin-3-yl)methanol can be compared with other similar compounds such as:
- Methoxy(pyridin-3-yl)methanol
- Ethoxy(pyridin-3-yl)methanol
- Propoxy(pyridin-3-yl)methanol
These compounds share a similar core structure but differ in the substituents attached to the methanol group. This compound is unique due to its specific functional groups, which confer distinct chemical and physical properties .
Conclusion
This compound is a versatile compound with significant applications in various fields of scientific research. Its unique chemical structure allows it to participate in diverse reactions, making it valuable in the synthesis of complex molecules and materials. Ongoing research continues to uncover new uses and potential benefits of this compound in chemistry, biology, medicine, and industry.
Properties
IUPAC Name |
dipyridin-3-ylmethanol | |
---|---|---|
Source | PubChem | |
URL | https://pubchem.ncbi.nlm.nih.gov | |
Description | Data deposited in or computed by PubChem | |
InChI |
InChI=1S/C11H10N2O/c14-11(9-3-1-5-12-7-9)10-4-2-6-13-8-10/h1-8,11,14H | |
Source | PubChem | |
URL | https://pubchem.ncbi.nlm.nih.gov | |
Description | Data deposited in or computed by PubChem | |
InChI Key |
DCDJTQNGTXDZGK-UHFFFAOYSA-N | |
Source | PubChem | |
URL | https://pubchem.ncbi.nlm.nih.gov | |
Description | Data deposited in or computed by PubChem | |
Canonical SMILES |
C1=CC(=CN=C1)C(C2=CN=CC=C2)O | |
Source | PubChem | |
URL | https://pubchem.ncbi.nlm.nih.gov | |
Description | Data deposited in or computed by PubChem | |
Molecular Formula |
C11H10N2O | |
Source | PubChem | |
URL | https://pubchem.ncbi.nlm.nih.gov | |
Description | Data deposited in or computed by PubChem | |
DSSTOX Substance ID |
DTXSID40461893 | |
Record name | Dipyridin-3-ylmethanol | |
Source | EPA DSSTox | |
URL | https://comptox.epa.gov/dashboard/DTXSID40461893 | |
Description | DSSTox provides a high quality public chemistry resource for supporting improved predictive toxicology. | |
Molecular Weight |
186.21 g/mol | |
Source | PubChem | |
URL | https://pubchem.ncbi.nlm.nih.gov | |
Description | Data deposited in or computed by PubChem | |
CAS No. |
89667-15-2 | |
Record name | Dipyridin-3-ylmethanol | |
Source | EPA DSSTox | |
URL | https://comptox.epa.gov/dashboard/DTXSID40461893 | |
Description | DSSTox provides a high quality public chemistry resource for supporting improved predictive toxicology. | |
Synthesis routes and methods I
Procedure details
Synthesis routes and methods II
Procedure details
Synthesis routes and methods III
Procedure details
Retrosynthesis Analysis
AI-Powered Synthesis Planning: Our tool employs the Template_relevance Pistachio, Template_relevance Bkms_metabolic, Template_relevance Pistachio_ringbreaker, Template_relevance Reaxys, Template_relevance Reaxys_biocatalysis model, leveraging a vast database of chemical reactions to predict feasible synthetic routes.
One-Step Synthesis Focus: Specifically designed for one-step synthesis, it provides concise and direct routes for your target compounds, streamlining the synthesis process.
Accurate Predictions: Utilizing the extensive PISTACHIO, BKMS_METABOLIC, PISTACHIO_RINGBREAKER, REAXYS, REAXYS_BIOCATALYSIS database, our tool offers high-accuracy predictions, reflecting the latest in chemical research and data.
Strategy Settings
Precursor scoring | Relevance Heuristic |
---|---|
Min. plausibility | 0.01 |
Model | Template_relevance |
Template Set | Pistachio/Bkms_metabolic/Pistachio_ringbreaker/Reaxys/Reaxys_biocatalysis |
Top-N result to add to graph | 6 |
Feasible Synthetic Routes
Disclaimer and Information on In-Vitro Research Products
Please be aware that all articles and product information presented on BenchChem are intended solely for informational purposes. The products available for purchase on BenchChem are specifically designed for in-vitro studies, which are conducted outside of living organisms. In-vitro studies, derived from the Latin term "in glass," involve experiments performed in controlled laboratory settings using cells or tissues. It is important to note that these products are not categorized as medicines or drugs, and they have not received approval from the FDA for the prevention, treatment, or cure of any medical condition, ailment, or disease. We must emphasize that any form of bodily introduction of these products into humans or animals is strictly prohibited by law. It is essential to adhere to these guidelines to ensure compliance with legal and ethical standards in research and experimentation.