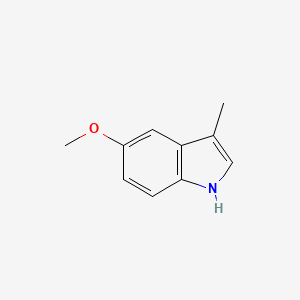
5-methoxy-3-methyl-1H-indole
Overview
Description
5-Methoxy-3-methyl-1H-indole is a naturally occurring compound belonging to the indole family, which are heterocyclic aromatic organic compounds Indoles are significant in various biological and chemical processes due to their structural similarity to tryptophan, an essential amino acid
Mechanism of Action
Target of Action
5-Methoxy-3-methyl-1H-indole, like many indole derivatives, has been found to bind with high affinity to multiple receptors . These receptors play a crucial role in various biological activities, including antiviral, anti-inflammatory, anticancer, anti-HIV, antioxidant, antimicrobial, antitubercular, antidiabetic, antimalarial, and anticholinesterase activities .
Mode of Action
Indole derivatives are known to interact with their targets, causing changes that result in their various biological activities . For example, some indole derivatives have been reported to show inhibitory activity against influenza A .
Biochemical Pathways
Indole derivatives are known to affect a wide range of biochemical pathways due to their broad-spectrum biological activities . For instance, some indole derivatives have been reported to inhibit the synthesis of certain proteins, affecting the pathways these proteins are involved in .
Pharmacokinetics
The lipophilicity and water solubility of indole derivatives can impact their bioavailability .
Result of Action
Indole derivatives are known to have various effects at the molecular and cellular level due to their diverse biological activities . For example, some indole derivatives have been reported to inhibit the growth of cancer cells .
Biochemical Analysis
Biochemical Properties
5-Methoxy-3-methyl-1H-indole interacts with various enzymes and proteins. It has been shown to inhibit the activity of myeloperoxidase (MPO), a peroxidase enzyme that plays a role in the immune response . The nature of this interaction involves the indole ring of the compound binding to the active site of the enzyme, thereby inhibiting its activity .
Cellular Effects
The effects of this compound on cells are diverse. It influences cell function by impacting cell signaling pathways, gene expression, and cellular metabolism . For instance, it has been found to inhibit the activity of MPO, which can affect the immune response of cells .
Molecular Mechanism
At the molecular level, this compound exerts its effects through various mechanisms. One such mechanism involves binding interactions with biomolecules, such as the inhibition of MPO . This interaction can lead to changes in gene expression and cellular metabolism .
Metabolic Pathways
This compound is involved in various metabolic pathways. It is used in the metabolic synthesis of arylacetic acid anti-inflammatory synthesis
Preparation Methods
Synthetic Routes and Reaction Conditions: The synthesis of 5-methoxy-3-methyl-1H-indole typically involves the Fischer indole synthesis, where an aryl hydrazine reacts with an aldehyde or ketone under acidic conditions. Another common method is the Biltz synthesis, which involves the cyclization of ortho-nitrotoluidine under high-temperature conditions.
Industrial Production Methods: On an industrial scale, the compound is often synthesized through continuous flow chemistry, which allows for better control over reaction conditions and improved safety. This method also enhances the scalability of the production process, making it more efficient and cost-effective.
Chemical Reactions Analysis
Types of Reactions: 5-Methoxy-3-methyl-1H-indole undergoes various chemical reactions, including oxidation, reduction, and substitution reactions.
Common Reagents and Conditions:
Oxidation: Common oxidizing agents include potassium permanganate (KMnO₄) and chromyl chloride (CrO₂Cl₂).
Reduction: Reduction reactions can be carried out using hydrogen gas (H₂) in the presence of a palladium catalyst.
Substitution: Nucleophilic substitution reactions often use strong bases like sodium hydride (NaH) or alkyl halides.
Major Products Formed:
Oxidation: Oxidation of this compound can yield various oxidized derivatives, such as this compound-2,3-dione.
Reduction: Reduction typically results in the formation of this compound-2-ethanamine.
Substitution: Substitution reactions can lead to the formation of alkylated derivatives, such as this compound-2-ethyl.
Scientific Research Applications
5-Methoxy-3-methyl-1H-indole has a wide range of applications in scientific research, including:
Chemistry: It serves as a building block for the synthesis of more complex organic compounds.
Biology: The compound has been studied for its potential biological activities, such as antimicrobial and antioxidant properties.
Medicine: It is being investigated for its potential therapeutic effects, including anti-inflammatory and anticancer activities.
Industry: It is used in the development of new materials and chemicals with unique properties.
Comparison with Similar Compounds
5-Methoxy-3-methyl-1H-indole is similar to other indole derivatives, such as 5-hydroxy-3-methyl-1H-indole and 5-methoxy-1H-indole. its unique methoxy and methyl groups contribute to its distinct chemical and biological properties. These differences can influence its reactivity, stability, and biological activity, making it a valuable compound for various applications.
Does this cover everything you were looking for, or is there something specific you'd like to dive deeper into?
Properties
IUPAC Name |
5-methoxy-3-methyl-1H-indole | |
---|---|---|
Source | PubChem | |
URL | https://pubchem.ncbi.nlm.nih.gov | |
Description | Data deposited in or computed by PubChem | |
InChI |
InChI=1S/C10H11NO/c1-7-6-11-10-4-3-8(12-2)5-9(7)10/h3-6,11H,1-2H3 | |
Source | PubChem | |
URL | https://pubchem.ncbi.nlm.nih.gov | |
Description | Data deposited in or computed by PubChem | |
InChI Key |
YSOFHURJVUIKMU-UHFFFAOYSA-N | |
Source | PubChem | |
URL | https://pubchem.ncbi.nlm.nih.gov | |
Description | Data deposited in or computed by PubChem | |
Canonical SMILES |
CC1=CNC2=C1C=C(C=C2)OC | |
Source | PubChem | |
URL | https://pubchem.ncbi.nlm.nih.gov | |
Description | Data deposited in or computed by PubChem | |
Molecular Formula |
C10H11NO | |
Source | PubChem | |
URL | https://pubchem.ncbi.nlm.nih.gov | |
Description | Data deposited in or computed by PubChem | |
DSSTOX Substance ID |
DTXSID70456499 | |
Record name | 5-methoxy-3-methyl-1H-indole | |
Source | EPA DSSTox | |
URL | https://comptox.epa.gov/dashboard/DTXSID70456499 | |
Description | DSSTox provides a high quality public chemistry resource for supporting improved predictive toxicology. | |
Molecular Weight |
161.20 g/mol | |
Source | PubChem | |
URL | https://pubchem.ncbi.nlm.nih.gov | |
Description | Data deposited in or computed by PubChem | |
CAS No. |
21987-25-7 | |
Record name | 5-methoxy-3-methyl-1H-indole | |
Source | EPA DSSTox | |
URL | https://comptox.epa.gov/dashboard/DTXSID70456499 | |
Description | DSSTox provides a high quality public chemistry resource for supporting improved predictive toxicology. | |
Synthesis routes and methods I
Procedure details
Synthesis routes and methods II
Procedure details
Retrosynthesis Analysis
AI-Powered Synthesis Planning: Our tool employs the Template_relevance Pistachio, Template_relevance Bkms_metabolic, Template_relevance Pistachio_ringbreaker, Template_relevance Reaxys, Template_relevance Reaxys_biocatalysis model, leveraging a vast database of chemical reactions to predict feasible synthetic routes.
One-Step Synthesis Focus: Specifically designed for one-step synthesis, it provides concise and direct routes for your target compounds, streamlining the synthesis process.
Accurate Predictions: Utilizing the extensive PISTACHIO, BKMS_METABOLIC, PISTACHIO_RINGBREAKER, REAXYS, REAXYS_BIOCATALYSIS database, our tool offers high-accuracy predictions, reflecting the latest in chemical research and data.
Strategy Settings
Precursor scoring | Relevance Heuristic |
---|---|
Min. plausibility | 0.01 |
Model | Template_relevance |
Template Set | Pistachio/Bkms_metabolic/Pistachio_ringbreaker/Reaxys/Reaxys_biocatalysis |
Top-N result to add to graph | 6 |
Feasible Synthetic Routes
Disclaimer and Information on In-Vitro Research Products
Please be aware that all articles and product information presented on BenchChem are intended solely for informational purposes. The products available for purchase on BenchChem are specifically designed for in-vitro studies, which are conducted outside of living organisms. In-vitro studies, derived from the Latin term "in glass," involve experiments performed in controlled laboratory settings using cells or tissues. It is important to note that these products are not categorized as medicines or drugs, and they have not received approval from the FDA for the prevention, treatment, or cure of any medical condition, ailment, or disease. We must emphasize that any form of bodily introduction of these products into humans or animals is strictly prohibited by law. It is essential to adhere to these guidelines to ensure compliance with legal and ethical standards in research and experimentation.