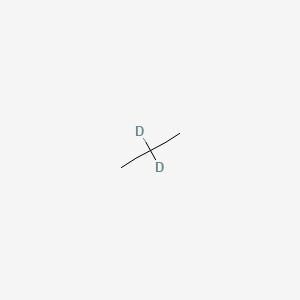
Propane-2,2-d2
Overview
Description
This compound is represented by the molecular formula CH3CD2CH3 and has a molecular weight of 46.11 g/mol . Deuterium is a heavier isotope of hydrogen, which makes deuterated compounds valuable in various scientific research applications, particularly in nuclear magnetic resonance (NMR) spectroscopy and mass spectrometry.
Preparation Methods
Synthetic Routes and Reaction Conditions
Propane-2,2-d2 can be synthesized through the deuteration of propane. One common method involves the reaction of propane with deuterium gas (D2) in the presence of a catalyst such as palladium on carbon (Pd/C) under high pressure and temperature conditions . Another method involves the use of deuterated reagents such as deuterated acetic acid (CD3COOD) to replace the hydrogen atoms with deuterium atoms .
Industrial Production Methods
Industrial production of this compound typically involves the large-scale deuteration of propane using deuterium gas. The process is carried out in specialized reactors designed to handle high pressures and temperatures. The deuterated propane is then purified through distillation and other separation techniques to achieve the desired isotopic purity .
Chemical Reactions Analysis
Types of Reactions
Propane-2,2-d2 undergoes similar chemical reactions as regular propane, including:
Combustion: this compound can undergo combustion in the presence of oxygen to produce carbon dioxide, water, and deuterium oxide (D2O).
Substitution: It can participate in substitution reactions where one or more hydrogen atoms are replaced by other atoms or groups.
Oxidation: this compound can be oxidized to form deuterated alcohols, aldehydes, and carboxylic acids.
Common Reagents and Conditions
Combustion: Requires oxygen and an ignition source.
Substitution: Common reagents include halogens (e.g., chlorine, bromine) and catalysts such as aluminum chloride (AlCl3).
Oxidation: Reagents such as potassium permanganate (KMnO4) or chromium trioxide (CrO3) are used under acidic or basic conditions.
Major Products Formed
Combustion: Carbon dioxide (CO2), water (H2O), and deuterium oxide (D2O).
Substitution: Deuterated halopropanes (e.g., CH3CD2CH2Cl).
Oxidation: Deuterated alcohols (e.g., CH3CD2OH), aldehydes (e.g., CH3CDO), and carboxylic acids (e.g., CD3COOH).
Scientific Research Applications
Propane-2,2-d2 is widely used in scientific research due to its unique properties:
Nuclear Magnetic Resonance (NMR) Spectroscopy: Deuterated compounds are used as solvents and internal standards in NMR spectroscopy to avoid interference from hydrogen signals.
Mass Spectrometry: Used as a reference compound to study fragmentation patterns and isotopic labeling.
Kinetic Isotope Effect Studies: Helps in understanding reaction mechanisms by comparing the rates of reactions involving hydrogen and deuterium.
Pharmaceutical Research: Used in the development of deuterated drugs to improve metabolic stability and reduce side effects.
Mechanism of Action
The mechanism of action of propane-2,2-d2 is primarily related to its use as a labeled compound in research. In NMR spectroscopy, the deuterium atoms provide distinct signals that help in the structural elucidation of molecules. In mass spectrometry, the presence of deuterium alters the fragmentation pattern, aiding in the identification of compounds. The kinetic isotope effect, where reactions involving deuterium proceed at different rates compared to hydrogen, provides insights into reaction mechanisms and pathways .
Comparison with Similar Compounds
Similar Compounds
Propane-1,1-d2: Another deuterated propane where the deuterium atoms are located at the first carbon.
Propane-1,2-d2: Deuterium atoms are located at the first and second carbons.
Propane-d8: Fully deuterated propane with all hydrogen atoms replaced by deuterium.
Uniqueness
Propane-2,2-d2 is unique due to the specific placement of deuterium atoms at the second carbon, which makes it particularly useful in studies involving the central carbon atom. This specific isotopic labeling provides distinct advantages in NMR spectroscopy and kinetic isotope effect studies compared to other deuterated propanes .
Properties
IUPAC Name |
2,2-dideuteriopropane | |
---|---|---|
Source | PubChem | |
URL | https://pubchem.ncbi.nlm.nih.gov | |
Description | Data deposited in or computed by PubChem | |
InChI |
InChI=1S/C3H8/c1-3-2/h3H2,1-2H3/i3D2 | |
Source | PubChem | |
URL | https://pubchem.ncbi.nlm.nih.gov | |
Description | Data deposited in or computed by PubChem | |
InChI Key |
ATUOYWHBWRKTHZ-SMZGMGDZSA-N | |
Source | PubChem | |
URL | https://pubchem.ncbi.nlm.nih.gov | |
Description | Data deposited in or computed by PubChem | |
Canonical SMILES |
CCC | |
Source | PubChem | |
URL | https://pubchem.ncbi.nlm.nih.gov | |
Description | Data deposited in or computed by PubChem | |
Isomeric SMILES |
[2H]C([2H])(C)C | |
Source | PubChem | |
URL | https://pubchem.ncbi.nlm.nih.gov | |
Description | Data deposited in or computed by PubChem | |
Molecular Formula |
C3H8 | |
Source | PubChem | |
URL | https://pubchem.ncbi.nlm.nih.gov | |
Description | Data deposited in or computed by PubChem | |
DSSTOX Substance ID |
DTXSID60426000 | |
Record name | Propane-2,2-d2 | |
Source | EPA DSSTox | |
URL | https://comptox.epa.gov/dashboard/DTXSID60426000 | |
Description | DSSTox provides a high quality public chemistry resource for supporting improved predictive toxicology. | |
Molecular Weight |
46.11 g/mol | |
Source | PubChem | |
URL | https://pubchem.ncbi.nlm.nih.gov | |
Description | Data deposited in or computed by PubChem | |
CAS No. |
2875-95-8 | |
Record name | Propane-2,2-d2 | |
Source | EPA DSSTox | |
URL | https://comptox.epa.gov/dashboard/DTXSID60426000 | |
Description | DSSTox provides a high quality public chemistry resource for supporting improved predictive toxicology. | |
Record name | 2875-95-8 | |
Source | European Chemicals Agency (ECHA) | |
URL | https://echa.europa.eu/information-on-chemicals | |
Description | The European Chemicals Agency (ECHA) is an agency of the European Union which is the driving force among regulatory authorities in implementing the EU's groundbreaking chemicals legislation for the benefit of human health and the environment as well as for innovation and competitiveness. | |
Explanation | Use of the information, documents and data from the ECHA website is subject to the terms and conditions of this Legal Notice, and subject to other binding limitations provided for under applicable law, the information, documents and data made available on the ECHA website may be reproduced, distributed and/or used, totally or in part, for non-commercial purposes provided that ECHA is acknowledged as the source: "Source: European Chemicals Agency, http://echa.europa.eu/". Such acknowledgement must be included in each copy of the material. ECHA permits and encourages organisations and individuals to create links to the ECHA website under the following cumulative conditions: Links can only be made to webpages that provide a link to the Legal Notice page. | |
Synthesis routes and methods I
Procedure details
Synthesis routes and methods II
Procedure details
Synthesis routes and methods III
Procedure details
Synthesis routes and methods IV
Procedure details
Retrosynthesis Analysis
AI-Powered Synthesis Planning: Our tool employs the Template_relevance Pistachio, Template_relevance Bkms_metabolic, Template_relevance Pistachio_ringbreaker, Template_relevance Reaxys, Template_relevance Reaxys_biocatalysis model, leveraging a vast database of chemical reactions to predict feasible synthetic routes.
One-Step Synthesis Focus: Specifically designed for one-step synthesis, it provides concise and direct routes for your target compounds, streamlining the synthesis process.
Accurate Predictions: Utilizing the extensive PISTACHIO, BKMS_METABOLIC, PISTACHIO_RINGBREAKER, REAXYS, REAXYS_BIOCATALYSIS database, our tool offers high-accuracy predictions, reflecting the latest in chemical research and data.
Strategy Settings
Precursor scoring | Relevance Heuristic |
---|---|
Min. plausibility | 0.01 |
Model | Template_relevance |
Template Set | Pistachio/Bkms_metabolic/Pistachio_ringbreaker/Reaxys/Reaxys_biocatalysis |
Top-N result to add to graph | 6 |
Feasible Synthetic Routes
Q1: How does the deuterium substitution in 2,2-dideuteriopropane affect its reactivity compared to propane?
A1: The deuterium isotope effect plays a significant role in the reactivity of 2,2-dideuteriopropane. Research using mercury-photosensitized reactions showed that ethylene doesn't undergo simple insertion reactions with 2,2-dideuteriopropane as readily as it does with propane. This suggests that the carbon-deuterium bond's higher bond dissociation energy influences the reaction pathway. [] Further studies investigating methylene insertion reactions revealed that methylene inserts into secondary carbon-hydrogen bonds in propane 1.23 times faster than into the carbon-deuterium bonds in 2,2-dideuteriopropane. [] This difference in reaction rates highlights the kinetic isotope effect, where the heavier deuterium slows down bond-breaking processes.
Q2: What insights did the study of 2,2-dideuteriopropane provide about the mechanism of methylene insertion reactions?
A2: The relatively small magnitude of the kinetic isotope effect observed in methylene insertion reactions with 2,2-dideuteriopropane provided evidence for a low activation energy in this reaction. [] This suggests that the insertion process is very efficient, proceeding rapidly once the reactants overcome a small energy barrier.
Q3: How is 2,2-dideuteriopropane used to understand radical reactions?
A3: 2,2-Dideuteriopropane serves as a valuable tool in studying the decomposition of radicals. By using propane-2,2-d2 in photolysis experiments with acetone-d6, researchers can track the fate of specific hydrogen (or deuterium) atoms and gain insights into the mechanism of n-propyl radical decomposition. []
Q4: Can you provide spectroscopic data for 2,2-dideuteriopropane?
A4: Carbon-13 Nuclear Magnetic Resonance (NMR) spectroscopy provides valuable structural information about 2,2-dideuteriopropane. Analysis of the carbon-13 NMR spectra of propane and this compound revealed a deuterium isotope shift at the C2 carbon of 0.72 ± 0.10 ppm to a higher field. [] This shift arises from the difference in nuclear spin and magnetic moment between hydrogen and deuterium, influencing the local magnetic environment experienced by the C2 carbon.
Q5: How is 2,2-dideuteriopropane used in studies of site-specific reactivity?
A5: Researchers used 2,2-dideuteriopropane, along with other deuterated propane and butane variants, to determine site-specific rate constants for hydrogen and deuterium abstraction by hydroxyl (OH) radicals. [] By comparing the reaction rates of OH with different deuterated isomers, they could isolate and quantify the reactivity of specific hydrogen atoms within the alkane molecules. This information is crucial for refining combustion models and understanding atmospheric chemistry processes.
Disclaimer and Information on In-Vitro Research Products
Please be aware that all articles and product information presented on BenchChem are intended solely for informational purposes. The products available for purchase on BenchChem are specifically designed for in-vitro studies, which are conducted outside of living organisms. In-vitro studies, derived from the Latin term "in glass," involve experiments performed in controlled laboratory settings using cells or tissues. It is important to note that these products are not categorized as medicines or drugs, and they have not received approval from the FDA for the prevention, treatment, or cure of any medical condition, ailment, or disease. We must emphasize that any form of bodily introduction of these products into humans or animals is strictly prohibited by law. It is essential to adhere to these guidelines to ensure compliance with legal and ethical standards in research and experimentation.