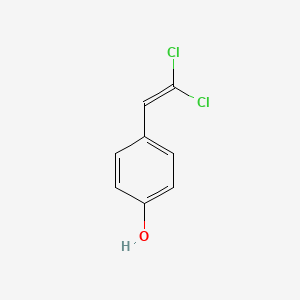
4-(2,2-dichloroethenyl)phenol
Overview
Description
4-(2,2-dichloroethenyl)phenol is an organic compound with the molecular formula C8H6Cl2O It consists of a phenol group substituted with a 2,2-dichloroethenyl group at the para position
Preparation Methods
Synthetic Routes and Reaction Conditions
4-(2,2-dichloroethenyl)phenol can be synthesized through nucleophilic aromatic substitution reactions. One common method involves the reaction of 4-chlorophenol with 1,1-dichloroethylene under basic conditions. The reaction typically requires a strong base such as sodium hydroxide and is carried out at elevated temperatures to facilitate the substitution reaction.
Industrial Production Methods
Industrial production of this compound may involve similar synthetic routes but on a larger scale. The process would be optimized for yield and purity, often involving continuous flow reactors and advanced purification techniques to ensure the final product meets industrial standards.
Chemical Reactions Analysis
Types of Reactions
4-(2,2-dichloroethenyl)phenol undergoes various chemical reactions, including:
Oxidation: The phenol group can be oxidized to form quinones.
Reduction: The dichloroethenyl group can be reduced to form ethyl derivatives.
Substitution: The compound can undergo further substitution reactions at the phenol ring.
Common Reagents and Conditions
Oxidation: Reagents such as potassium permanganate or chromium trioxide in acidic conditions.
Reduction: Catalytic hydrogenation using palladium on carbon or other hydrogenation catalysts.
Substitution: Strong bases like sodium hydroxide or potassium tert-butoxide in polar aprotic solvents.
Major Products Formed
Oxidation: Formation of quinones.
Reduction: Formation of ethyl derivatives.
Substitution: Various substituted phenols depending on the substituent introduced.
Scientific Research Applications
4-(2,2-dichloroethenyl)phenol has several applications in scientific research:
Chemistry: Used as an intermediate in the synthesis of more complex organic molecules.
Biology: Studied for its potential effects on biological systems, including its role as a ligand in biochemical assays.
Medicine: Investigated for its potential therapeutic properties, including antimicrobial and anticancer activities.
Industry: Utilized in the production of specialty chemicals and materials.
Mechanism of Action
The mechanism of action of 4-(2,2-dichloroethenyl)phenol involves its interaction with molecular targets such as enzymes and receptors. The phenol group can form hydrogen bonds with active sites, while the dichloroethenyl group can participate in hydrophobic interactions. These interactions can modulate the activity of the target molecules, leading to various biological effects.
Comparison with Similar Compounds
Similar Compounds
- 2,4-dichlorophenol
- 4-chlorophenol
- 2,2-dichloroethanol
Uniqueness
4-(2,2-dichloroethenyl)phenol is unique due to the presence of both a phenol group and a dichloroethenyl group, which confer distinct chemical reactivity and biological activity. Compared to similar compounds, it offers a unique combination of properties that make it valuable for specific applications in research and industry.
Properties
CAS No. |
83671-20-9 |
---|---|
Molecular Formula |
C8H6Cl2O |
Molecular Weight |
189.04 g/mol |
IUPAC Name |
4-(2,2-dichloroethenyl)phenol |
InChI |
InChI=1S/C8H6Cl2O/c9-8(10)5-6-1-3-7(11)4-2-6/h1-5,11H |
InChI Key |
DQQXPDAVLLJOGV-UHFFFAOYSA-N |
SMILES |
C1=CC(=CC=C1C=C(Cl)Cl)O |
Canonical SMILES |
C1=CC(=CC=C1C=C(Cl)Cl)O |
Origin of Product |
United States |
Synthesis routes and methods I
Procedure details
Synthesis routes and methods II
Procedure details
Disclaimer and Information on In-Vitro Research Products
Please be aware that all articles and product information presented on BenchChem are intended solely for informational purposes. The products available for purchase on BenchChem are specifically designed for in-vitro studies, which are conducted outside of living organisms. In-vitro studies, derived from the Latin term "in glass," involve experiments performed in controlled laboratory settings using cells or tissues. It is important to note that these products are not categorized as medicines or drugs, and they have not received approval from the FDA for the prevention, treatment, or cure of any medical condition, ailment, or disease. We must emphasize that any form of bodily introduction of these products into humans or animals is strictly prohibited by law. It is essential to adhere to these guidelines to ensure compliance with legal and ethical standards in research and experimentation.