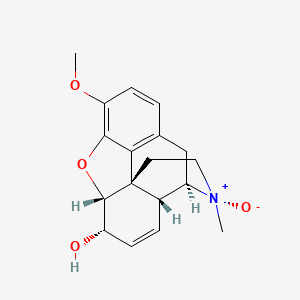
Codeine N-oxide
Overview
Description
Preparation Methods
Synthetic Routes and Reaction Conditions: Codeine-N-oxide can be synthesized through the oxidation of codeine. One common method involves the use of m-chloroperbenzoic acid as the oxidizing agent. The reaction is typically carried out in chloroform at low temperatures (0 ± 5°C) to ensure controlled oxidation .
Industrial Production Methods: Industrial production of Codeine-N-oxide follows similar synthetic routes but on a larger scale. The process involves careful control of reaction conditions to ensure high yield and purity of the product. The use of advanced purification techniques such as column chromatography is common to isolate the desired compound .
Types of Reactions:
Oxidation: Codeine-N-oxide is formed through the oxidation of codeine.
Reduction: It can undergo reduction reactions to revert to codeine.
Substitution: Various substitution reactions can occur at different positions on the molecule.
Common Reagents and Conditions:
Oxidation: m-Chloroperbenzoic acid in chloroform at low temperatures.
Reduction: Hydrogenation using palladium on carbon as a catalyst.
Substitution: Halogenation using halogenating agents like bromine or chlorine.
Major Products:
Oxidation: Codeine-N-oxide.
Reduction: Codeine.
Substitution: Halogenated derivatives of Codeine-N-oxide.
Scientific Research Applications
Pharmacological Applications
1. Anticancer Properties:
Recent studies have demonstrated the potential anticancer effects of codeine N-oxide. A study published in the Journal of the Chilean Chemical Society indicated that codeine and its complexes exhibited significant cytotoxicity against various cancer cell lines, including MCF-7 (breast cancer) and AGS (gastric cancer) cells. The results showed that these compounds reduced viable cell numbers in a dose-dependent manner, suggesting their potential use as natural anticancer agents .
2. Antimicrobial Activity:
this compound has also been investigated for its antibacterial properties. Research indicated that both codeine and its complexes displayed inhibitory effects against several bacterial strains, including Pseudomonas aeruginosa and Staphylococcus aureus. The study utilized MTT assays to evaluate cytotoxic effects and found that the ligand and its synthetic complexes exhibited significant antimicrobial activity .
Analytical Applications
1. Detection Methods:
The determination of this compound in biological samples has become increasingly important due to its pharmacological relevance. Various analytical methods have been developed for this purpose:
- Electrochemical Detection: Recent advancements include the use of aptamer-based sensors combined with differential pulse voltammetry (DPV), achieving remarkably low detection limits for this compound .
- Chromatographic Techniques: High-performance liquid chromatography (HPLC) has been employed to analyze the pharmacokinetics of codeine derivatives, including N-oxides, providing insights into their metabolism and clearance rates in human subjects .
Case Studies and Research Findings
1. Case Study on Cancer Treatment:
A notable case study highlighted the efficacy of codeine complexes in inhibiting cancer cell proliferation. The combination of codeine with iron(III) complexes showed enhanced cytotoxicity compared to codeine alone, indicating a synergistic effect that could be leveraged in cancer therapies .
2. Safety and Efficacy Reviews:
The Therapeutic Goods Administration (TGA) has conducted extensive reviews on the safety profiles of codeine-containing products, including those involving this compound. These reviews emphasize the importance of understanding individual metabolic responses to codeine, particularly in populations with varying CYP2D6 enzyme activity, which affects the conversion of codeine to its active forms .
Mechanism of Action
Codeine-N-oxide exerts its effects by binding to the mu-opioid receptors in the central nervous system. This binding leads to hyperpolarization of neurons, resulting in the inhibition of nociceptive neurotransmitter release. This mechanism causes an analgesic effect and increases pain tolerance by reducing neuronal excitability .
Comparison with Similar Compounds
Morphine-N-oxide: Another nitrogen derivative of morphine, similar in structure and function to Codeine-N-oxide.
Hydromorphone-N-oxide: A nitrogen derivative of hydromorphone, also studied for its pharmaceutical potential.
Comparison:
Codeine-N-oxide vs. Morphine-N-oxide: Both are oxidation products of their parent compounds, but Codeine-N-oxide is derived from codeine, while Morphine-N-oxide is derived from morphine. Codeine-N-oxide is weaker in analgesic effect compared to Morphine-N-oxide.
Codeine-N-oxide vs. Hydromorphone-N-oxide: Both are nitrogen derivatives, but Hydromorphone-N-oxide is derived from hydromorphone and is generally more potent than Codeine-N-oxide.
Biological Activity
Codeine N-oxide is an oxidized derivative of codeine, a well-known opioid analgesic. This compound has garnered attention due to its potential biological activities, which include antitumor effects, antimicrobial properties, and implications in pharmacokinetics. This article reviews the current understanding of the biological activity of this compound, supported by research findings and case studies.
This compound is formed through the oxidation of codeine, a process that can occur during drug metabolism or as a degradation product in pharmaceutical formulations. The presence of this compound can influence the pharmacological profile of codeine, altering its efficacy and safety.
Antitumor Activity
Recent studies have demonstrated that this compound exhibits significant antitumor activity against various cancer cell lines.
Case Study: Anticancer Properties
A study investigated the cytotoxic effects of this compound on AGS (stomach) and MCF-7 (breast) cancer cell lines. The results indicated that:
- Dose-Dependent Inhibition : this compound reduced the viability of both AGS and MCF-7 cells in a dose-dependent manner.
- IC50 Values : The IC50 value for AGS cells was determined to be 10 mmol, while for MCF-7 cells it was 2.5 mmol after 72 hours of incubation .
Cell Line | IC50 Value (mmol) | Time (hours) |
---|---|---|
AGS | 10 | 72 |
MCF-7 | 2.5 | 72 |
This suggests that this compound could potentially serve as an effective agent in cancer therapies, particularly when combined with other compounds to enhance its efficacy.
Antimicrobial Activity
In addition to its anticancer properties, this compound has been studied for its antimicrobial activity .
In-Vitro Studies
The antimicrobial efficacy was assessed through inhibition zone measurements against various microbial strains. The results indicated:
- Inhibition zones greater than 5 mm were considered indicative of antimicrobial activity.
Complex | Inhibition Zone (mm) |
---|---|
This compound | >5 |
These findings suggest that this compound may possess potential as an antimicrobial agent, although further research is needed to elucidate its mechanism of action.
Pharmacokinetics and Metabolism
Understanding the pharmacokinetics of this compound is crucial for evaluating its therapeutic potential.
Study on Pharmacokinetics
A pharmacokinetic study involving healthy Greyhound dogs administered IV codeine revealed important insights:
- Half-Life : The half-life of codeine was approximately 1.22 hours.
- Bioavailability : Oral bioavailability was found to be only about 4%, indicating significant first-pass metabolism.
The study also highlighted that large amounts of codeine-6-glucuronide were produced, suggesting that metabolic pathways significantly influence the pharmacological effects of codeine and its derivatives .
Safety and Toxicity Considerations
Despite its potential benefits, the use of this compound raises safety concerns, particularly regarding its metabolism to morphine in ultra-rapid metabolizers.
Case Reports
Reports indicate that individuals who metabolize codeine rapidly may experience severe respiratory depression due to increased morphine levels, emphasizing the need for careful monitoring in clinical settings .
Chemical Reactions Analysis
Formation via Oxidation
Codeine N-oxide is synthesized through the oxidation of codeine. Hydrogen peroxide (H₂O₂) is a common oxidizing agent for this reaction, though the process may involve trace intermediates or competing hydroxylation pathways. For example:
-
In a study analyzing codeine’s reaction with H₂O₂, minor products with m/z 316 (consistent with this compound’s molecular weight) were detected via LC/MS, though structural confirmation was limited .
-
General N-oxide synthesis involves oxidizing tertiary amines (e.g., codeine’s nitrogen) using peracids or H₂O₂ under controlled conditions .
Reaction Pathway:
Reduction Reactions
This compound can revert to codeine under reductive conditions, a key feature in prodrug activation. This process is catalyzed by reductases, particularly in hypoxic environments:
-
Hypoxia-activated prodrugs (HAPs) like tirapazamine undergo one-electron reduction via cytochrome P450 enzymes, generating reactive radicals .
-
This compound’s reduction likely involves similar mechanisms, releasing codeine and forming transient electrophilic intermediates .
Table 1: Reductive Activation of this compound
Condition | Catalyst | Product | Application |
---|---|---|---|
Hypoxia | Cytochrome P450 | Codeine + Electrophile | Targeted drug release |
Chemical reduction | Stannous chloride/HCl | Codeine | Synthetic reversal |
Thermal Decomposition
Aromatic N-oxides decompose at elevated temperatures (~150°C) via syn-elimination or rearrangements:
-
Polonovski Reaction : Involves N-oxide acylation, leading to dealkylation (e.g., demethylation of this compound) .
Example Reaction (Polonovski):
Reactivity with Electrophiles
N-oxides react violently with carbodiimides (e.g., DCC, EDC), posing safety risks in industrial settings . For this compound:
-
Residual carbodiimides in cellulose manufacturing (Lyocell process) could trigger autocatalytic decomposition .
Radical and Electron Transfer Reactions
N-oxides participate in single-electron transfer processes:
-
Pyridine-N-oxides mimic tyrosine radicals in artificial photosynthesis .
-
This compound may exhibit antioxidant properties through radical scavenging .
Hydrogen Bonding and Stability
The N⁺–O⁻ bond in this compound facilitates strong hydrogen bonding, stabilizing carbonyl hydrates in oxidation reactions . This property is exploited in catalytic protocols (e.g., TPAP-mediated alcohol oxidations) .
Analytical Challenges
-
This compound and hydroxylated codeine derivatives (e.g., hydroxycodeine) share similar m/z values, complicating LC/MS differentiation .
-
Structural confirmation requires advanced techniques like tandem MS/MS or NMR .
Pharmacological Implications
Q & A
Basic Research Questions
Q. What synthetic methods are used to prepare Codeine N-oxide, and how is its purity validated?
this compound is synthesized via N-oxidation of codeine using oxidizing agents such as meta-chloroperbenzoic acid (mCPBA) under controlled conditions. Post-synthesis, thin-layer chromatography (TLC) and gas-liquid chromatography (GLC) are employed to separate and validate the compound against known standards. For purity assessment, nuclear magnetic resonance (NMR) and high-performance liquid chromatography (HPLC) are used to confirm structural integrity and quantify impurities. New compounds require full spectral characterization, while known compounds should cross-reference published retention times and Rf values .
Q. How does the metabolic activity of this compound compare to its parent compound, codeine?
this compound retains pharmacological activity similar to codeine, as observed in tertiary amine N-oxide metabolites (e.g., imipramine N-oxide). Metabolic studies involve in vitro models (e.g., liver microsomes) to track biotransformation pathways, including reduction back to codeine. Comparative assays measure receptor binding affinity and metabolic stability using techniques like liquid chromatography-mass spectrometry (LC-MS) to quantify parent and metabolite concentrations over time .
Q. What analytical techniques are recommended for identifying this compound in complex biological matrices?
TLC and GLC remain foundational for preliminary identification due to their cost-effectiveness and reproducibility. For higher sensitivity, LC-MS or LC-tandem MS is preferred, especially in biological fluids. Calibration with certified reference materials and internal standards (e.g., deuterated analogs) ensures accuracy. Method validation should follow ICH guidelines, including tests for linearity, precision, and matrix effects .
Advanced Research Questions
Q. How do environmental factors (pH, temperature) influence the stability of this compound in aqueous solutions?
Degradation kinetics are studied under varying pH (1–13) and temperature (4–40°C) conditions. Samples are analyzed at intervals using UV-Vis spectroscopy or HPLC to monitor decomposition products (e.g., codeine or norcodeine). Buffering agents and counter-ion effects must be controlled, as they may accelerate isomerization or hydrolysis. Data is modeled using Arrhenius equations to predict shelf-life and storage requirements .
Q. What methodologies are used to assess the mutagenic or antigenotoxic potential of this compound?
The Ames test (with Salmonella strains TA98 and TA100) evaluates mutagenicity by measuring reverse mutations in the presence of metabolic activation (S9 fraction). Antigenotoxicity studies involve co-treatment with known mutagens (e.g., 2-aminoanthracene) and quantifying mutation suppression via the fluctuation assay. Positive controls and dose-response curves are critical to validate results .
Q. How can contradictory data in metabolic studies of this compound be resolved?
Contradictions often arise from interspecies differences in enzyme expression (e.g., CYP450 isoforms). To address this, researchers should:
- Replicate experiments across multiple models (e.g., human vs. rodent microsomes).
- Use siRNA knockdown or chemical inhibitors to isolate specific metabolic pathways.
- Apply multivariate statistical analysis to distinguish experimental noise from biologically significant variation .
Q. What strategies ensure reproducibility in this compound synthesis across laboratories?
Detailed protocols must include:
- Exact molar ratios of reactants and solvents.
- Reaction time/temperature profiles.
- Chromatographic parameters (e.g., column type, mobile phase).
- Raw spectral data (NMR, MS) in supplementary materials.
Collaborative trials across labs and open-access data sharing (e.g., via repositories like Zenodo) enhance reproducibility .
Q. How do this compound’s physicochemical properties compare to other opioid N-oxides, and what implications does this have for drug design?
Comparative studies use computational tools (e.g., molecular docking, DFT calculations) to assess LogP, pKa, and binding affinity to opioid receptors. In vitro permeability assays (Caco-2 cells) and in vivo pharmacokinetic profiling (rodents) evaluate bioavailability. Structural analogs (e.g., morphine N-oxide) serve as benchmarks to identify structure-activity relationships .
Properties
CAS No. |
3688-65-1 |
---|---|
Molecular Formula |
C18H21NO4 |
Molecular Weight |
315.4 g/mol |
IUPAC Name |
(4R,4aR,7S,7aR,12bS)-9-methoxy-3-methyl-3-oxido-2,4,4a,7,7a,13-hexahydro-1H-4,12-methanobenzofuro[3,2-e]isoquinolin-3-ium-7-ol |
InChI |
InChI=1S/C18H21NO4/c1-19(21)8-7-18-11-4-5-13(20)17(18)23-16-14(22-2)6-3-10(15(16)18)9-12(11)19/h3-6,11-13,17,20H,7-9H2,1-2H3/t11-,12+,13-,17-,18-,19?/m0/s1 |
InChI Key |
BDLSDHWCOJPHIE-YMVRPXFZSA-N |
SMILES |
C[N+]1(CCC23C4C1CC5=C2C(=C(C=C5)OC)OC3C(C=C4)O)[O-] |
Isomeric SMILES |
C[N+]1(CC[C@]23[C@@H]4[C@H]1CC5=C2C(=C(C=C5)OC)O[C@H]3[C@H](C=C4)O)[O-] |
Canonical SMILES |
C[N+]1(CCC23C4C1CC5=C2C(=C(C=C5)OC)OC3C(C=C4)O)[O-] |
melting_point |
231 - 232 °C |
Key on ui other cas no. |
3688-65-1 |
physical_description |
Solid |
Pictograms |
Acute Toxic; Irritant; Health Hazard |
Origin of Product |
United States |
Retrosynthesis Analysis
AI-Powered Synthesis Planning: Our tool employs the Template_relevance Pistachio, Template_relevance Bkms_metabolic, Template_relevance Pistachio_ringbreaker, Template_relevance Reaxys, Template_relevance Reaxys_biocatalysis model, leveraging a vast database of chemical reactions to predict feasible synthetic routes.
One-Step Synthesis Focus: Specifically designed for one-step synthesis, it provides concise and direct routes for your target compounds, streamlining the synthesis process.
Accurate Predictions: Utilizing the extensive PISTACHIO, BKMS_METABOLIC, PISTACHIO_RINGBREAKER, REAXYS, REAXYS_BIOCATALYSIS database, our tool offers high-accuracy predictions, reflecting the latest in chemical research and data.
Strategy Settings
Precursor scoring | Relevance Heuristic |
---|---|
Min. plausibility | 0.01 |
Model | Template_relevance |
Template Set | Pistachio/Bkms_metabolic/Pistachio_ringbreaker/Reaxys/Reaxys_biocatalysis |
Top-N result to add to graph | 6 |
Feasible Synthetic Routes
Disclaimer and Information on In-Vitro Research Products
Please be aware that all articles and product information presented on BenchChem are intended solely for informational purposes. The products available for purchase on BenchChem are specifically designed for in-vitro studies, which are conducted outside of living organisms. In-vitro studies, derived from the Latin term "in glass," involve experiments performed in controlled laboratory settings using cells or tissues. It is important to note that these products are not categorized as medicines or drugs, and they have not received approval from the FDA for the prevention, treatment, or cure of any medical condition, ailment, or disease. We must emphasize that any form of bodily introduction of these products into humans or animals is strictly prohibited by law. It is essential to adhere to these guidelines to ensure compliance with legal and ethical standards in research and experimentation.