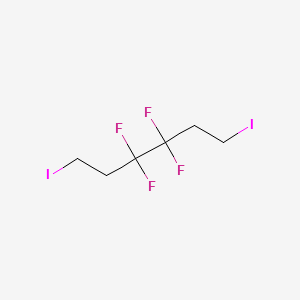
1,6-Diiodo-3,3,4,4-tetrafluorohexane
Overview
Description
1,6-Diiodo-3,3,4,4-tetrafluorohexane is a chemical compound with the molecular formula C6H8F4I2 and a molecular weight of 409.93 g/mol . It is characterized by the presence of four fluorine atoms and two iodine atoms attached to a hexane backbone. This compound is primarily used in scientific research and industrial applications due to its unique chemical properties.
Preparation Methods
Synthetic Routes and Reaction Conditions
The synthesis of 3,3,4,4-tetrafluoro-1,6-diiodohexane typically involves the reaction of ethylene with 1,2-diiodotetrafluoroethane . The reaction conditions often require a controlled environment with specific temperature and pressure settings to ensure the desired product is obtained. The process may involve the use of catalysts to facilitate the reaction and improve yield.
Industrial Production Methods
Industrial production of 3,3,4,4-tetrafluoro-1,6-diiodohexane follows similar synthetic routes but on a larger scale. The process is optimized for efficiency and cost-effectiveness, often involving continuous flow reactors and automated systems to maintain consistent quality and high production rates .
Chemical Reactions Analysis
Types of Reactions
1,6-Diiodo-3,3,4,4-tetrafluorohexane undergoes various chemical reactions, including:
Substitution Reactions: The iodine atoms can be substituted with other functional groups using appropriate reagents.
Reduction Reactions: The compound can be reduced to form different derivatives.
Oxidation Reactions: Oxidation can lead to the formation of new compounds with different properties.
Common Reagents and Conditions
Substitution: Reagents such as sodium iodide in acetone can be used for halogen exchange reactions.
Reduction: Reducing agents like lithium aluminum hydride (LiAlH4) are commonly used.
Oxidation: Oxidizing agents such as potassium permanganate (KMnO4) can be employed.
Major Products Formed
The major products formed from these reactions depend on the specific reagents and conditions used. For example, substitution reactions can yield fluorinated derivatives, while reduction reactions can produce hydrocarbon chains with varying degrees of fluorination .
Scientific Research Applications
1,6-Diiodo-3,3,4,4-tetrafluorohexane has several applications in scientific research:
Polymer Synthesis: It is used in the synthesis of fluorinated polymers, which possess high thermal and chemical stability, low surface energy, and excellent weather resistance.
Coatings and Films: Due to its low surface energy, it is used in coatings for non-stick cookware, self-cleaning surfaces, and protective films for electronic devices.
Mechanism of Action
The mechanism of action of 3,3,4,4-tetrafluoro-1,6-diiodohexane involves its interaction with molecular targets through its fluorine and iodine atoms. These interactions can lead to changes in the chemical and physical properties of the target molecules, enabling the compound to exert its effects. The specific pathways involved depend on the application and the nature of the target molecules .
Comparison with Similar Compounds
Similar Compounds
- 1,2-Bis(2-iodoethyl)tetrafluoroethane
- 2,2,3,3-Tetrafluorobutane-1,4-diyl dicarbamate
- 2,2,3,3-Tetrafluorobutane-1,4-diyl dinitrate
Uniqueness
1,6-Diiodo-3,3,4,4-tetrafluorohexane is unique due to its specific arrangement of fluorine and iodine atoms on a hexane backbone. This structure imparts distinct chemical properties, such as high reactivity in substitution reactions and stability in various environmental conditions .
Properties
IUPAC Name |
3,3,4,4-tetrafluoro-1,6-diiodohexane | |
---|---|---|
Source | PubChem | |
URL | https://pubchem.ncbi.nlm.nih.gov | |
Description | Data deposited in or computed by PubChem | |
InChI |
InChI=1S/C6H8F4I2/c7-5(8,1-3-11)6(9,10)2-4-12/h1-4H2 | |
Source | PubChem | |
URL | https://pubchem.ncbi.nlm.nih.gov | |
Description | Data deposited in or computed by PubChem | |
InChI Key |
JFYZKVSETQEPKR-UHFFFAOYSA-N | |
Source | PubChem | |
URL | https://pubchem.ncbi.nlm.nih.gov | |
Description | Data deposited in or computed by PubChem | |
Canonical SMILES |
C(CI)C(C(CCI)(F)F)(F)F | |
Source | PubChem | |
URL | https://pubchem.ncbi.nlm.nih.gov | |
Description | Data deposited in or computed by PubChem | |
Molecular Formula |
C6H8F4I2 | |
Source | PubChem | |
URL | https://pubchem.ncbi.nlm.nih.gov | |
Description | Data deposited in or computed by PubChem | |
DSSTOX Substance ID |
DTXSID90382165 | |
Record name | 3,3,4,4-tetrafluoro-1,6-diiodohexane | |
Source | EPA DSSTox | |
URL | https://comptox.epa.gov/dashboard/DTXSID90382165 | |
Description | DSSTox provides a high quality public chemistry resource for supporting improved predictive toxicology. | |
Molecular Weight |
409.93 g/mol | |
Source | PubChem | |
URL | https://pubchem.ncbi.nlm.nih.gov | |
Description | Data deposited in or computed by PubChem | |
CAS No. |
2163-06-6 | |
Record name | 3,3,4,4-tetrafluoro-1,6-diiodohexane | |
Source | EPA DSSTox | |
URL | https://comptox.epa.gov/dashboard/DTXSID90382165 | |
Description | DSSTox provides a high quality public chemistry resource for supporting improved predictive toxicology. | |
Record name | 1,6-Diiodo-3,3,4,4-tetrafluorohexane | |
Source | European Chemicals Agency (ECHA) | |
URL | https://echa.europa.eu/information-on-chemicals | |
Description | The European Chemicals Agency (ECHA) is an agency of the European Union which is the driving force among regulatory authorities in implementing the EU's groundbreaking chemicals legislation for the benefit of human health and the environment as well as for innovation and competitiveness. | |
Explanation | Use of the information, documents and data from the ECHA website is subject to the terms and conditions of this Legal Notice, and subject to other binding limitations provided for under applicable law, the information, documents and data made available on the ECHA website may be reproduced, distributed and/or used, totally or in part, for non-commercial purposes provided that ECHA is acknowledged as the source: "Source: European Chemicals Agency, http://echa.europa.eu/". Such acknowledgement must be included in each copy of the material. ECHA permits and encourages organisations and individuals to create links to the ECHA website under the following cumulative conditions: Links can only be made to webpages that provide a link to the Legal Notice page. | |
Retrosynthesis Analysis
AI-Powered Synthesis Planning: Our tool employs the Template_relevance Pistachio, Template_relevance Bkms_metabolic, Template_relevance Pistachio_ringbreaker, Template_relevance Reaxys, Template_relevance Reaxys_biocatalysis model, leveraging a vast database of chemical reactions to predict feasible synthetic routes.
One-Step Synthesis Focus: Specifically designed for one-step synthesis, it provides concise and direct routes for your target compounds, streamlining the synthesis process.
Accurate Predictions: Utilizing the extensive PISTACHIO, BKMS_METABOLIC, PISTACHIO_RINGBREAKER, REAXYS, REAXYS_BIOCATALYSIS database, our tool offers high-accuracy predictions, reflecting the latest in chemical research and data.
Strategy Settings
Precursor scoring | Relevance Heuristic |
---|---|
Min. plausibility | 0.01 |
Model | Template_relevance |
Template Set | Pistachio/Bkms_metabolic/Pistachio_ringbreaker/Reaxys/Reaxys_biocatalysis |
Top-N result to add to graph | 6 |
Feasible Synthetic Routes
Disclaimer and Information on In-Vitro Research Products
Please be aware that all articles and product information presented on BenchChem are intended solely for informational purposes. The products available for purchase on BenchChem are specifically designed for in-vitro studies, which are conducted outside of living organisms. In-vitro studies, derived from the Latin term "in glass," involve experiments performed in controlled laboratory settings using cells or tissues. It is important to note that these products are not categorized as medicines or drugs, and they have not received approval from the FDA for the prevention, treatment, or cure of any medical condition, ailment, or disease. We must emphasize that any form of bodily introduction of these products into humans or animals is strictly prohibited by law. It is essential to adhere to these guidelines to ensure compliance with legal and ethical standards in research and experimentation.