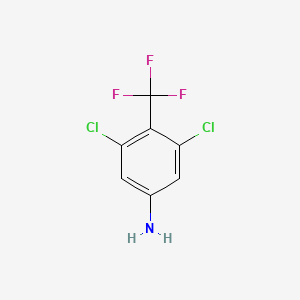
3,5-Dichloro-4-(trifluoromethyl)aniline
Overview
Description
3,5-Dichloro-4-(trifluoromethyl)aniline: is an organic compound with the molecular formula C7H4Cl2F3N . It is a derivative of aniline, where the hydrogen atoms on the benzene ring are substituted with chlorine and trifluoromethyl groups. This compound is known for its applications in various fields, including pharmaceuticals, agrochemicals, and material sciences .
Mechanism of Action
Target of Action
3,5-Dichloro-4-(trifluoromethyl)aniline is a key intermediate in the synthesis of Hexaflumuron , a type of benzoylphenylurea insecticide . Hexaflumuron can inhibit the synthesis of chitin in target pests, leading to their death or infertility .
Mode of Action
The compound is used in the Suzuki–Miyaura (SM) cross-coupling reaction, a widely applied transition metal catalyzed carbon–carbon bond forming reaction . In this reaction, oxidative addition occurs with formally electrophilic organic groups, whereby palladium becomes oxidized through its donation of electrons to form the new Pd–C bond . Transmetalation occurs with formally nucleophilic organic groups, which are transferred from boron to palladium .
Biochemical Pathways
The compound is involved in the Suzuki–Miyaura cross-coupling reaction, which is a key step in the synthesis of many organic compounds . This reaction is part of the broader field of palladium-catalyzed coupling reactions, which are fundamental processes in organic chemistry.
Pharmacokinetics
The compound’s properties such as density, boiling point, and molecular weight have been reported . These properties can influence the compound’s Absorption, Distribution, Metabolism, and Excretion (ADME) properties, and thus its bioavailability.
Result of Action
The primary result of the action of this compound is the formation of new carbon-carbon bonds via the Suzuki–Miyaura cross-coupling reaction . This allows for the synthesis of complex organic compounds, including pharmaceuticals and agrochemicals.
Action Environment
The action of this compound can be influenced by various environmental factors. For example, the Suzuki–Miyaura cross-coupling reaction requires specific reaction conditions, including the presence of a palladium catalyst and an organoboron reagent . Additionally, the reaction is sensitive to the concentration of ammonia .
Biochemical Analysis
Biochemical Properties
3,5-Dichloro-4-(trifluoromethyl)aniline plays a significant role in biochemical reactions, particularly in the inhibition of specific enzymes. It interacts with enzymes such as cytochrome P450, which is involved in the metabolism of various substances. The compound binds to the active site of the enzyme, leading to inhibition of its activity. This interaction can affect the metabolism of other compounds that are substrates for cytochrome P450 .
Cellular Effects
The effects of this compound on cells are profound. It has been observed to influence cell signaling pathways, gene expression, and cellular metabolism. The compound can induce oxidative stress in cells, leading to the activation of stress-responsive signaling pathways. This can result in changes in gene expression, particularly those genes involved in antioxidant defense mechanisms . Additionally, this compound can disrupt cellular metabolism by inhibiting key metabolic enzymes, leading to altered energy production and utilization .
Molecular Mechanism
At the molecular level, this compound exerts its effects through binding interactions with biomolecules. It can bind to the active sites of enzymes, leading to inhibition or activation of their activity. For instance, the compound inhibits cytochrome P450 by binding to its heme group, preventing the enzyme from metabolizing its substrates . This inhibition can lead to the accumulation of toxic metabolites and oxidative stress in cells .
Temporal Effects in Laboratory Settings
In laboratory settings, the effects of this compound change over time. The compound is relatively stable under standard laboratory conditions, but it can degrade over extended periods. Studies have shown that prolonged exposure to the compound can lead to long-term effects on cellular function, including persistent oxidative stress and altered gene expression . These effects are more pronounced in in vitro studies, where cells are continuously exposed to the compound .
Dosage Effects in Animal Models
The effects of this compound vary with different dosages in animal models. At low doses, the compound may have minimal effects on cellular function. At higher doses, it can induce significant toxicity, including liver and kidney damage . Threshold effects have been observed, where a certain dosage level leads to a sudden increase in adverse effects. Toxic effects at high doses include oxidative stress, inflammation, and apoptosis in various tissues .
Metabolic Pathways
This compound is involved in several metabolic pathways. It is primarily metabolized by cytochrome P450 enzymes, which convert it into various metabolites. These metabolites can further interact with other enzymes and cofactors, affecting metabolic flux and metabolite levels . The compound’s metabolism can lead to the production of reactive oxygen species, contributing to oxidative stress in cells .
Transport and Distribution
Within cells and tissues, this compound is transported and distributed through various mechanisms. It can interact with transporters and binding proteins, facilitating its movement across cellular membranes . The compound tends to accumulate in specific tissues, such as the liver and kidneys, where it exerts its toxic effects . Its distribution is influenced by factors such as lipid solubility and binding affinity to cellular components .
Subcellular Localization
The subcellular localization of this compound affects its activity and function. The compound can localize to specific organelles, such as the mitochondria and endoplasmic reticulum, where it interacts with enzymes and other biomolecules . Targeting signals and post-translational modifications can direct the compound to these compartments, influencing its biochemical effects . The localization to mitochondria, for example, can lead to mitochondrial dysfunction and oxidative stress .
Preparation Methods
Synthetic Routes and Reaction Conditions: The synthesis of 3,5-Dichloro-4-(trifluoromethyl)aniline typically involves the chlorination and fluorination of precursor compounds. One common method starts with p-Chlorobenzotrifluoride , which undergoes ring chlorination to form 3,4,5-Trichlorobenzotrifluoride and 3,4-Dichlorobenzotrifluoride . The latter is then subjected to fluoridization and ammoniation reactions to yield this compound .
Industrial Production Methods: Industrial production of this compound often employs similar synthetic routes but on a larger scale, with optimized reaction conditions to ensure high yield and purity. The use of catalysts and controlled reaction environments is common to enhance efficiency and reduce by-products .
Chemical Reactions Analysis
Types of Reactions: 3,5-Dichloro-4-(trifluoromethyl)aniline can undergo various chemical reactions, including:
Substitution Reactions: The chlorine atoms can be substituted with other functional groups under appropriate conditions.
Oxidation and Reduction Reactions: The compound can be oxidized or reduced to form different derivatives.
Coupling Reactions: It can participate in coupling reactions, such as Suzuki–Miyaura coupling, to form more complex molecules.
Common Reagents and Conditions:
Substitution Reactions: Reagents like sodium hydroxide or potassium carbonate in polar solvents.
Oxidation Reactions: Oxidizing agents like potassium permanganate or hydrogen peroxide.
Coupling Reactions: Palladium catalysts and organoboron reagents under mild conditions.
Major Products: The major products formed from these reactions depend on the specific reagents and conditions used. For example, substitution reactions can yield various substituted anilines, while coupling reactions can produce biaryl compounds .
Scientific Research Applications
Chemistry: In chemistry, 3,5-Dichloro-4-(trifluoromethyl)aniline is used as a building block for synthesizing more complex molecules. Its unique structure makes it valuable in the development of new materials and catalysts .
Biology and Medicine: The compound is explored for its potential biological activities. Derivatives of this compound are investigated for their antimicrobial, antifungal, and anticancer properties .
Industry: In the industrial sector, this compound is used in the synthesis of agrochemicals, such as pesticides and herbicides. It is also employed in the production of specialty chemicals and advanced materials .
Comparison with Similar Compounds
- 2,6-Dichloro-4-(trifluoromethyl)aniline
- 3,5-Dichloro-4-(1,1,2,2-tetrafluoroethoxy)aniline
- 4-Amino-3,5-dichlorobenzotrifluoride
Comparison: Compared to its similar compounds, 3,5-Dichloro-4-(trifluoromethyl)aniline is unique due to its specific substitution pattern on the benzene ring. This unique structure can result in different chemical reactivity and biological activity, making it suitable for specific applications where other compounds may not be as effective .
Properties
IUPAC Name |
3,5-dichloro-4-(trifluoromethyl)aniline | |
---|---|---|
Source | PubChem | |
URL | https://pubchem.ncbi.nlm.nih.gov | |
Description | Data deposited in or computed by PubChem | |
InChI |
InChI=1S/C7H4Cl2F3N/c8-4-1-3(13)2-5(9)6(4)7(10,11)12/h1-2H,13H2 | |
Source | PubChem | |
URL | https://pubchem.ncbi.nlm.nih.gov | |
Description | Data deposited in or computed by PubChem | |
InChI Key |
LOVGLWZIYWMBLT-UHFFFAOYSA-N | |
Source | PubChem | |
URL | https://pubchem.ncbi.nlm.nih.gov | |
Description | Data deposited in or computed by PubChem | |
Canonical SMILES |
C1=C(C=C(C(=C1Cl)C(F)(F)F)Cl)N | |
Source | PubChem | |
URL | https://pubchem.ncbi.nlm.nih.gov | |
Description | Data deposited in or computed by PubChem | |
Molecular Formula |
C7H4Cl2F3N | |
Source | PubChem | |
URL | https://pubchem.ncbi.nlm.nih.gov | |
Description | Data deposited in or computed by PubChem | |
DSSTOX Substance ID |
DTXSID60381261 | |
Record name | 3,5-dichloro-4-(trifluoromethyl)aniline | |
Source | EPA DSSTox | |
URL | https://comptox.epa.gov/dashboard/DTXSID60381261 | |
Description | DSSTox provides a high quality public chemistry resource for supporting improved predictive toxicology. | |
Molecular Weight |
230.01 g/mol | |
Source | PubChem | |
URL | https://pubchem.ncbi.nlm.nih.gov | |
Description | Data deposited in or computed by PubChem | |
CAS No. |
496052-55-2 | |
Record name | 3,5-dichloro-4-(trifluoromethyl)aniline | |
Source | EPA DSSTox | |
URL | https://comptox.epa.gov/dashboard/DTXSID60381261 | |
Description | DSSTox provides a high quality public chemistry resource for supporting improved predictive toxicology. | |
Retrosynthesis Analysis
AI-Powered Synthesis Planning: Our tool employs the Template_relevance Pistachio, Template_relevance Bkms_metabolic, Template_relevance Pistachio_ringbreaker, Template_relevance Reaxys, Template_relevance Reaxys_biocatalysis model, leveraging a vast database of chemical reactions to predict feasible synthetic routes.
One-Step Synthesis Focus: Specifically designed for one-step synthesis, it provides concise and direct routes for your target compounds, streamlining the synthesis process.
Accurate Predictions: Utilizing the extensive PISTACHIO, BKMS_METABOLIC, PISTACHIO_RINGBREAKER, REAXYS, REAXYS_BIOCATALYSIS database, our tool offers high-accuracy predictions, reflecting the latest in chemical research and data.
Strategy Settings
Precursor scoring | Relevance Heuristic |
---|---|
Min. plausibility | 0.01 |
Model | Template_relevance |
Template Set | Pistachio/Bkms_metabolic/Pistachio_ringbreaker/Reaxys/Reaxys_biocatalysis |
Top-N result to add to graph | 6 |
Feasible Synthetic Routes
Disclaimer and Information on In-Vitro Research Products
Please be aware that all articles and product information presented on BenchChem are intended solely for informational purposes. The products available for purchase on BenchChem are specifically designed for in-vitro studies, which are conducted outside of living organisms. In-vitro studies, derived from the Latin term "in glass," involve experiments performed in controlled laboratory settings using cells or tissues. It is important to note that these products are not categorized as medicines or drugs, and they have not received approval from the FDA for the prevention, treatment, or cure of any medical condition, ailment, or disease. We must emphasize that any form of bodily introduction of these products into humans or animals is strictly prohibited by law. It is essential to adhere to these guidelines to ensure compliance with legal and ethical standards in research and experimentation.