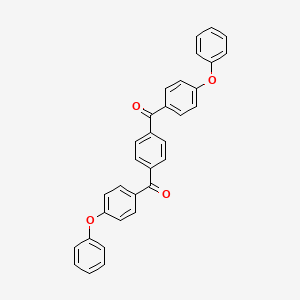
1,4-Bis(4-phenoxybenzoyl)benzene
Overview
Description
1,4-Bis(4-phenoxybenzoyl)benzene (CAS 54299-17-1; molecular formula C₃₂H₂₂O₄, molecular weight 470.51 g/mol) is a high-performance aromatic poly(aryl ether ketone) (PAEK) derivative. It is synthesized via nucleophilic substitution of 1,4-bis(4-chlorobenzoyl)benzene with sodium phenoxide or through Friedel-Crafts acylation of terephthaloyl chloride with diphenyl ether . The compound exhibits a crystalline structure similar to polyetherketoneketone (PEKK), with distinct X-ray diffraction (XRD) peaks at 2θ = 18.5°, 22.8°, and 28.5° . Its thermal stability (melting point: 211–217°C) and chemical resistance make it suitable for advanced engineering plastics, coatings, and high-temperature composites .
Preparation Methods
General Synthetic Route
The core synthetic approach to 1,4-bis(4-phenoxybenzoyl)benzene involves the reaction of terephthaloyl chloride (TPC) with diphenyl ether (DPO) catalyzed by a Lewis acid, typically aluminum chloride (AlCl3), in an organic solvent such as ortho-dichlorobenzene. This reaction proceeds via a Friedel-Crafts acylation mechanism, producing the target compound predominantly as a complex with the Lewis acid catalyst.
- Formation of an active intermediate, 4-(4-phenoxybenzoyl)benzoyl chloride, by reaction of one molecule of terephthaloyl chloride with one molecule of diphenyl ether.
- Subsequent acylation leads to the formation of this compound.
- Workup involves neutralization and separation of catalyst and by-products.
Optimized Reaction Conditions and Process
A recent European patent (EP 4206176 A1) describes an improved preparation method emphasizing temperature control to reduce energy consumption and allow solvent reuse without degradation.
Step | Description | Conditions |
---|---|---|
(a) | Dissolution of terephthaloyl chloride and diphenyl ether in solvent (e.g., ortho-dichlorobenzene) | Ambient temperature |
(b) | Cooling of solution | -10°C to -5°C |
(c) | Addition of Lewis acid catalyst (AlCl3) and synthesis reaction by warming | Raise temperature to 20°C–40°C |
(d) | Cooling post-reaction and catalyst desorption | 1°C to 15°C, methanol addition |
(e) | Filtration and separation of product and waste solvent | Ambient to low temperature |
- Performing the synthesis reaction at 20°C to 30°C significantly reduces heating and cooling energy compared to higher temperatures (40°C or 60°C).
- The yield of this compound remains consistent across these temperature ranges.
- Waste solvent after neutralization exhibits minimal color change (APHA 1–10, Yellow Index 0.1–1), indicating its suitability for reuse, contributing to energy and cost savings.
Comparative Energy and Yield Analysis
Experimental data comparing synthesis at different temperatures demonstrate the benefits of controlled temperature reaction:
Parameter | Example 1 (20°C) | Example 2 (30°C) | Comp. Example 1 (40°C) | Comp. Example 2 (60°C) |
---|---|---|---|---|
Reaction Temperature (°C) | 20 | 30 | 40 | 60 |
Heating and Cooling Energy | Lowest | Low | Higher | Highest |
Product Yield | High | High | Comparable | Comparable |
Waste Solvent APHA Value | 1–10 | 1–10 | Higher | Higher |
Waste Solvent Yellow Index | 0.1–1 | 0.1–1 | Higher | Higher |
Solvent Reuse Feasibility | High | High | Low | Low |
These results confirm that maintaining synthesis between 20°C and 30°C optimizes energy efficiency without compromising product yield, and enhances solvent recyclability.
Catalyst and Solvent Considerations
- Catalyst: Aluminum chloride is the preferred Lewis acid catalyst, facilitating the Friedel-Crafts acylation efficiently.
- Solvent: Ortho-dichlorobenzene is commonly used due to its ability to dissolve reactants and withstand reaction conditions.
- Post-reaction treatment: Methanol is used for catalyst desorption and facilitates separation of the product from the catalyst and solvent.
Summary of Preparation Method Advantages
- Energy efficiency: Controlled temperature synthesis reduces heating/cooling demands.
- High yield: Maintains consistent high yield of this compound.
- Solvent reuse: Waste solvent remains clear and color-stable after neutralization, allowing recycling.
- Scalability: The method is suitable for industrial-scale production with improved environmental and economic profiles.
Detailed Research Findings
- The reaction proceeds via a two-stage mechanism involving an active intermediate (4-(4-phenoxybenzoyl)benzoyl chloride).
- Temperature control is critical to minimize side reactions and catalyst degradation.
- Neutralization of waste solvent with NaOH removes residual AlCl3 and particulate matter.
- Colorimetric analysis (APHA and Yellow Index) serves as a quality metric for solvent reuse potential.
- The method aligns with green chemistry principles by reducing waste and energy consumption.
Chemical Reactions Analysis
Types of Reactions: 1,4-Bis(4-phenoxybenzoyl)benzene undergoes various chemical reactions, including:
Oxidation: This compound can be oxidized using strong oxidizing agents such as potassium permanganate (KMnO({3})).
Reduction: Reduction reactions can be carried out using reducing agents like lithium aluminum hydride (LiAlH({4})).
Substitution: Electrophilic and nucleophilic substitution reactions are possible, depending on the reagents and conditions used.
Common Reagents and Conditions:
Oxidation: KMnO(_{4}) in acidic or basic medium.
Reduction: LiAlH({4}) in methanol.
Substitution: Halogenation using bromine (Br({2})) in the presence of a catalyst.
Major Products Formed:
Oxidation: Formation of carboxylic acids or ketones.
Reduction: Formation of alcohols or alkanes.
Substitution: Formation of halogenated derivatives.
Scientific Research Applications
Chemical Applications
Building Block in Organic Synthesis
1,4-Bis(4-phenoxybenzoyl)benzene serves as a crucial building block for synthesizing complex organic molecules and polymers. The compound can be utilized in various chemical reactions, such as Friedel-Crafts acylation, which allows for the introduction of acyl groups into aromatic compounds. This property makes it valuable in producing specialty chemicals and advanced materials.
Polymer Production
The compound is integral to synthesizing polyether ketone (PEK) and polyether ketone ketone (PEKK), high-performance polymers known for their thermal stability and mechanical strength. These polymers are applicable in aerospace, automotive, and medical devices due to their resistance to high temperatures and chemical environments .
Biological Applications
Investigations in Drug Delivery
Research has indicated potential uses of this compound in drug delivery systems. Its ability to form complexes with biological macromolecules may enhance the solubility and bioavailability of pharmaceutical compounds. Studies are ongoing to explore its efficacy as a carrier for targeted drug delivery .
Proteomics Research
The compound is also used in proteomics research as a biochemical tool. It aids in the study of protein interactions and functions, contributing to advancements in understanding cellular processes and disease mechanisms .
Industrial Applications
Production of High-Performance Materials
In industrial settings, this compound is utilized to produce heat-resistant coatings and materials. Its incorporation into formulations enhances the thermal stability and durability of products used in extreme conditions, such as those found in aerospace applications .
Energy-Efficient Manufacturing Processes
Recent innovations have focused on improving the synthesis methods for this compound to minimize energy consumption. For instance, methods that allow for solvent reuse and reduced heating requirements have been developed, enhancing the sustainability of its production .
Case Study 1: Synthesis Optimization
A study demonstrated that by controlling the synthesis temperature during the reaction of terephthaloyl chloride with diphenyl ether using aluminum chloride as a catalyst, researchers could maintain high yields while reducing energy input. This method not only improved efficiency but also minimized waste generation .
Case Study 2: Drug Delivery Systems
In a series of experiments aimed at developing novel drug delivery systems, researchers explored the interaction between this compound and various therapeutic agents. Results indicated enhanced solubility profiles for certain drugs when complexed with this compound, suggesting its potential role in improving drug formulation strategies .
Data Tables
Application Area | Specific Use Case | Benefits |
---|---|---|
Chemistry | Synthesis of complex organic molecules | Versatile building block |
Polymer Science | Production of PEK/PEKK | High thermal stability |
Medicine | Drug delivery systems | Improved solubility and bioavailability |
Industrial Coatings | Heat-resistant materials | Enhanced durability under extreme conditions |
Energy Efficiency | Optimized synthesis methods | Reduced energy consumption and waste generation |
Mechanism of Action
The mechanism by which 1,4-Bis(4-phenoxybenzoyl)benzene exerts its effects depends on its specific application. In chemical reactions, its reactivity is influenced by the electron-withdrawing nature of the benzoyl groups, which can stabilize reaction intermediates. In biological systems, it may interact with proteins or nucleic acids through non-covalent interactions, such as hydrogen bonding or π-π stacking.
Comparison with Similar Compounds
Comparison with Structurally Similar Compounds
The following table summarizes key properties of 1,4-bis(4-phenoxybenzoyl)benzene and related compounds:
Key Comparisons :
Thermal Stability: this compound (211–217°C) outperforms 1,4-bis(4-methylstyryl)benzene (decomposes above 250°C) and 1,4-bis(trichloromethyl)benzene (106–110°C) due to its rigid aromatic backbone and ketone linkages .
Optical Properties: 1,4-Bis(4-methylstyryl)benzene nanocrystals exhibit fluorescence emission at 450 nm (quantum yield: ~35%), making them superior for photonic applications compared to the non-fluorescent this compound .
Chemical Reactivity: The chlorine-rich 1,4-bis(trichloromethyl)benzene is highly reactive and toxic, whereas this compound’s phenoxy groups enhance oxidative stability .
Biological Activity: Thiazolo-oxadiazole derivatives (e.g., 4,4′-Bis[(2″-arylthiazolo-oxadiazolyl) bibenzyl]) show fungicidal activity absent in this compound, highlighting the impact of heterocyclic substituents .
Crystallinity: this compound shares XRD patterns with PEKK, while 1,4-bis(4-aminophenoxy)benzene forms 1D hydrogen-bonded chains, demonstrating how functional groups dictate supramolecular assembly .
Biological Activity
1,4-Bis(4-phenoxybenzoyl)benzene is an organic compound characterized by its unique structure, comprising a benzene ring substituted with two 4-phenoxybenzoyl groups. Its molecular formula is and it has garnered attention for its potential biological activities, particularly in the fields of medicinal chemistry and materials science.
The biological activity of this compound is primarily attributed to its ability to form Lewis acid complexes. This property is significant in various chemical reactions and biological interactions. The compound has been investigated for its potential as an anticancer agent, where it acts on topoisomerase I, an enzyme critical for DNA replication and transcription. This interaction suggests that the compound may inhibit cancer cell proliferation by disrupting DNA processes .
Toxicity Profile
The toxicity assessment of this compound indicates that it has a relatively low toxicity profile. In animal studies:
- Oral LD50 (rat) : >2000 mg/kg
- Dermal LD50 (rat) : >2000 mg/kg
- Inhalation LC50 (rat) : >5.33 mg/L over 4 hours
These results suggest that while the compound can cause irritation to the eyes and skin, it does not pose significant chronic health risks under controlled exposure conditions .
Anticancer Activity
Research has highlighted the compound's potential as an anticancer drug. A study indicated that this compound exhibits selective cytotoxicity towards cancer cells while sparing normal cells. The mechanism involves the inhibition of topoisomerase I, leading to DNA damage and apoptosis in cancer cells. This finding positions the compound as a candidate for further development in cancer therapy .
Polymer Science Applications
In polymer science, this compound serves as a precursor for high-performance polymers due to its thermal stability and mechanical properties. It is used in the synthesis of polyether ketones which are valued for their strength and resistance to heat and chemicals.
Comparative Analysis with Related Compounds
To understand the unique properties of this compound, it is useful to compare it with similar compounds:
Compound | Structure Variation | Biological Activity |
---|---|---|
1,4-Bis(4-methoxybenzoyl)benzene | Methoxy groups instead of phenoxy | Lower solubility in organic solvents |
1,4-Bis(4-chlorobenzoyl)benzene | Chlorine atoms | Increased reactivity |
1,4-Bis(4-nitrobenzoyl)benzene | Nitro groups | Different electronic properties |
This table illustrates how variations in substituents can influence the solubility, reactivity, and ultimately the biological activity of these compounds.
Q & A
Basic Research Questions
Q. What are the standard synthetic routes for 1,4-Bis(4-phenoxybenzoyl)benzene, and how do reaction conditions influence yield and purity?
The compound is synthesized via Friedel-Crafts acylation, where 1,4-diphenoxybenzene reacts with 4-phenoxybenzoyl chloride in the presence of a Lewis acid catalyst (e.g., AlCl₃). Key parameters include solvent choice (e.g., dichloromethane), temperature (0–25°C), and stoichiometric ratios. Post-synthesis purification typically involves recrystallization from high-boiling solvents like dimethylformamide (DMF) to achieve >99% purity . Yield optimization requires careful control of moisture, as hydrolyzed intermediates can form side products.
Q. Which spectroscopic and crystallographic techniques are most effective for characterizing this compound?
- NMR : ¹H/¹³C NMR confirms aromatic proton environments and carbonyl linkages.
- FTIR : Peaks at ~1650 cm⁻¹ (C=O stretching) and 1240 cm⁻¹ (C-O-C ether linkages) validate the structure.
- XRD : Single-crystal X-ray diffraction reveals a planar backbone with dihedral angles <10° between benzene rings, influencing π-π stacking behavior.
- DSC/TGA : Melting point (211–217°C) and thermal stability (decomposition >400°C) are critical for polymer applications .
Q. How can researchers address discrepancies in reported melting points or solubility data?
Variations in melting points (e.g., 211–217°C vs. narrower ranges) often arise from polymorphic forms or residual solvents. Differential Scanning Calorimetry (DSC) with controlled heating rates (e.g., 10°C/min) under nitrogen can identify polymorphs. Solubility inconsistencies in polar aprotic solvents (DMF, DMSO) may require standardized saturation protocols (e.g., sonication at 25°C for 24 hours) .
Advanced Research Questions
Q. What mechanistic insights explain the compound’s role in poly(arylene ether ketone) synthesis via Friedel-Crafts polymerization?
The electron-rich phenoxy groups activate the benzene ring for electrophilic substitution, while the ketone linkages stabilize the growing polymer chain. Computational studies (DFT/B3LYP) show that the planar structure lowers activation energy (~15 kJ/mol) for acylation compared to non-planar analogs. Chain propagation is influenced by steric hindrance from the 4-phenoxy substituents, requiring excess monomer to achieve high molecular weights (>50 kDa) .
Q. How do computational models predict the electronic and optical properties of this compound?
Hartree-Fock (HF) and MP2 methods with 6-31G** basis sets accurately calculate dipole moments (~3.5 D) and polarizabilities (α = 450 a.u.). The extended conjugation results in a low HOMO-LUMO gap (~4.1 eV), suggesting potential as a semiconductor. Nonlinear optical properties (hyperpolarizability β ~1.2 × 10⁻³⁰ esu) are enhanced by electron-withdrawing ketone groups, making it suitable for photonic applications .
Q. What photochemical degradation pathways are plausible under UV irradiation, and how can stability be improved?
Analogous to 1,4-bis(phenylsulfonyloxy)benzene, UV exposure (254 nm) may induce radical formation via ketone cleavage. ESR studies detect triplet-state intermediates, leading to Fries rearrangement or crosslinking. Stabilization strategies include incorporating UV absorbers (e.g., hindered amine light stabilizers) or synthesizing derivatives with electron-donating substituents (e.g., methoxy groups) to reduce radical generation .
Q. How does the compound’s structure influence its performance in metal-organic frameworks (MOFs) or supramolecular assemblies?
The rigid, planar structure enables π-stacking with aromatic ligands (e.g., pyridyl groups), forming 2D MOFs with pore sizes ~1.2 nm. Coordination with transition metals (e.g., Cu²⁺) via ketone oxygen atoms enhances thermal stability (>500°C). In supramolecular gels, van der Waals interactions between phenoxy groups facilitate self-assembly in nonpolar solvents .
Q. Data Contradiction Analysis
Q. Why do computational and experimental dipole moments sometimes diverge, and how can this be resolved?
Discrepancies arise from solvent effects (e.g., polar solvents stabilize charge-separated states) and approximations in basis sets. Hybrid methods like CAM-B3LYP with implicit solvation models (e.g., PCM) reduce errors to <5%. Experimental validation via Stark spectroscopy in thin films is recommended .
Q. What factors explain inconsistent polymer molecular weights reported in Friedel-Crafts polymerization?
Variability stems from catalyst purity (AlCl₃ vs. FeCl₃), moisture content, and monomer feeding rates. Kinetic studies show that stepwise addition of 4-phenoxybenzoyl chloride minimizes oligomerization. Size-exclusion chromatography (SEC) with triple detection (RI/UV/light scattering) provides accurate molecular weight distributions .
Q. Methodological Recommendations
- Synthesis : Use anhydrous AlCl₃ in dichloromethane under argon for reproducible yields.
- Characterization : Combine XRD with Hirshfeld surface analysis to assess crystal packing efficiency.
- Computational Studies : Employ MP2/6-311+G(d,p) for higher accuracy in hyperpolarizability calculations.
Properties
IUPAC Name |
[4-(4-phenoxybenzoyl)phenyl]-(4-phenoxyphenyl)methanone | |
---|---|---|
Source | PubChem | |
URL | https://pubchem.ncbi.nlm.nih.gov | |
Description | Data deposited in or computed by PubChem | |
InChI |
InChI=1S/C32H22O4/c33-31(25-15-19-29(20-16-25)35-27-7-3-1-4-8-27)23-11-13-24(14-12-23)32(34)26-17-21-30(22-18-26)36-28-9-5-2-6-10-28/h1-22H | |
Source | PubChem | |
URL | https://pubchem.ncbi.nlm.nih.gov | |
Description | Data deposited in or computed by PubChem | |
InChI Key |
NWJVKSITTJQWCG-UHFFFAOYSA-N | |
Source | PubChem | |
URL | https://pubchem.ncbi.nlm.nih.gov | |
Description | Data deposited in or computed by PubChem | |
Canonical SMILES |
C1=CC=C(C=C1)OC2=CC=C(C=C2)C(=O)C3=CC=C(C=C3)C(=O)C4=CC=C(C=C4)OC5=CC=CC=C5 | |
Source | PubChem | |
URL | https://pubchem.ncbi.nlm.nih.gov | |
Description | Data deposited in or computed by PubChem | |
Molecular Formula |
C32H22O4 | |
Source | PubChem | |
URL | https://pubchem.ncbi.nlm.nih.gov | |
Description | Data deposited in or computed by PubChem | |
DSSTOX Substance ID |
DTXSID5074056 | |
Record name | Methanone, 1,4-phenylenebis[(4-phenoxyphenyl)- | |
Source | EPA DSSTox | |
URL | https://comptox.epa.gov/dashboard/DTXSID5074056 | |
Description | DSSTox provides a high quality public chemistry resource for supporting improved predictive toxicology. | |
Molecular Weight |
470.5 g/mol | |
Source | PubChem | |
URL | https://pubchem.ncbi.nlm.nih.gov | |
Description | Data deposited in or computed by PubChem | |
CAS No. |
54299-17-1 | |
Record name | 1,1′-(1,4-Phenylene)bis[1-(4-phenoxyphenyl)methanone] | |
Source | CAS Common Chemistry | |
URL | https://commonchemistry.cas.org/detail?cas_rn=54299-17-1 | |
Description | CAS Common Chemistry is an open community resource for accessing chemical information. Nearly 500,000 chemical substances from CAS REGISTRY cover areas of community interest, including common and frequently regulated chemicals, and those relevant to high school and undergraduate chemistry classes. This chemical information, curated by our expert scientists, is provided in alignment with our mission as a division of the American Chemical Society. | |
Explanation | The data from CAS Common Chemistry is provided under a CC-BY-NC 4.0 license, unless otherwise stated. | |
Record name | Methanone, 1,4-phenylenebis((4-phenoxyphenyl)- | |
Source | ChemIDplus | |
URL | https://pubchem.ncbi.nlm.nih.gov/substance/?source=chemidplus&sourceid=0054299171 | |
Description | ChemIDplus is a free, web search system that provides access to the structure and nomenclature authority files used for the identification of chemical substances cited in National Library of Medicine (NLM) databases, including the TOXNET system. | |
Record name | Methanone, 1,4-phenylenebis[(4-phenoxyphenyl)- | |
Source | EPA DSSTox | |
URL | https://comptox.epa.gov/dashboard/DTXSID5074056 | |
Description | DSSTox provides a high quality public chemistry resource for supporting improved predictive toxicology. | |
Record name | 1,4-phenylenebis[(4-phenoxyphenyl)methanone] | |
Source | European Chemicals Agency (ECHA) | |
URL | https://echa.europa.eu/substance-information/-/substanceinfo/100.124.437 | |
Description | The European Chemicals Agency (ECHA) is an agency of the European Union which is the driving force among regulatory authorities in implementing the EU's groundbreaking chemicals legislation for the benefit of human health and the environment as well as for innovation and competitiveness. | |
Explanation | Use of the information, documents and data from the ECHA website is subject to the terms and conditions of this Legal Notice, and subject to other binding limitations provided for under applicable law, the information, documents and data made available on the ECHA website may be reproduced, distributed and/or used, totally or in part, for non-commercial purposes provided that ECHA is acknowledged as the source: "Source: European Chemicals Agency, http://echa.europa.eu/". Such acknowledgement must be included in each copy of the material. ECHA permits and encourages organisations and individuals to create links to the ECHA website under the following cumulative conditions: Links can only be made to webpages that provide a link to the Legal Notice page. | |
Retrosynthesis Analysis
AI-Powered Synthesis Planning: Our tool employs the Template_relevance Pistachio, Template_relevance Bkms_metabolic, Template_relevance Pistachio_ringbreaker, Template_relevance Reaxys, Template_relevance Reaxys_biocatalysis model, leveraging a vast database of chemical reactions to predict feasible synthetic routes.
One-Step Synthesis Focus: Specifically designed for one-step synthesis, it provides concise and direct routes for your target compounds, streamlining the synthesis process.
Accurate Predictions: Utilizing the extensive PISTACHIO, BKMS_METABOLIC, PISTACHIO_RINGBREAKER, REAXYS, REAXYS_BIOCATALYSIS database, our tool offers high-accuracy predictions, reflecting the latest in chemical research and data.
Strategy Settings
Precursor scoring | Relevance Heuristic |
---|---|
Min. plausibility | 0.01 |
Model | Template_relevance |
Template Set | Pistachio/Bkms_metabolic/Pistachio_ringbreaker/Reaxys/Reaxys_biocatalysis |
Top-N result to add to graph | 6 |
Feasible Synthetic Routes
Disclaimer and Information on In-Vitro Research Products
Please be aware that all articles and product information presented on BenchChem are intended solely for informational purposes. The products available for purchase on BenchChem are specifically designed for in-vitro studies, which are conducted outside of living organisms. In-vitro studies, derived from the Latin term "in glass," involve experiments performed in controlled laboratory settings using cells or tissues. It is important to note that these products are not categorized as medicines or drugs, and they have not received approval from the FDA for the prevention, treatment, or cure of any medical condition, ailment, or disease. We must emphasize that any form of bodily introduction of these products into humans or animals is strictly prohibited by law. It is essential to adhere to these guidelines to ensure compliance with legal and ethical standards in research and experimentation.