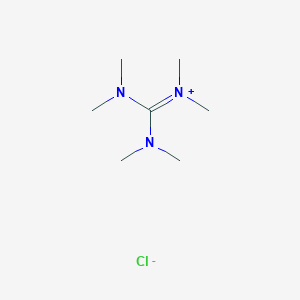
Hexamethylguanidinium chloride
Overview
Description
Hexamethylguanidinium chloride is an organic compound with the molecular formula C₇H₁₈ClN₃. It is a white crystalline powder known for its high melting point of 286-287°C . This compound is a derivative of guanidine, where all hydrogen atoms are replaced by methyl groups, resulting in a highly symmetrical and stable structure.
Mechanism of Action
Target of Action
Hexamethylguanidinium chloride (HMG Cl) is primarily used as a phase-transfer catalyst . It has been found to perform exceptionally well in high-temperature fluoride chloride halex exchange . The compound’s primary targets are the reactants in this exchange process, facilitating their interaction and promoting the reaction.
Mode of Action
It is known that the compound interacts with its targets by acting as a catalyst, reducing the energy barrier for the reaction and thus accelerating the reaction rate .
Biochemical Pathways
The biochemical pathways affected by HMG Cl are those involved in the fluoride chloride halex exchange . This reaction is crucial in many industrial processes, and the role of HMG Cl as a catalyst can significantly enhance the efficiency of these processes.
Result of Action
The primary result of HMG Cl’s action is the facilitation of the fluoride chloride halex exchange . By acting as a catalyst, HMG Cl can significantly increase the rate of this reaction, leading to increased efficiency in industrial processes that rely on this exchange.
Biochemical Analysis
Biochemical Properties
Hexamethylguanidinium chloride plays a significant role in biochemical reactions. It interacts with various enzymes, proteins, and other biomolecules. One of the key interactions is with guanidinium-rich molecules, which facilitate cellular translocation and delivery of bioactive cargos through biological barriers . The compound’s guanidinium groups are essential for binding interactions with cell surface counterparts, enabling efficient cellular uptake . Additionally, this compound has been shown to influence the structural, physical, and thermal properties of organic ionic plastic crystals, making it a promising material for electrochemical energy storage technologies .
Cellular Effects
This compound has notable effects on various types of cells and cellular processes. It influences cell function by facilitating the uptake of bioactive molecules, which can impact cell signaling pathways, gene expression, and cellular metabolism . The compound’s ability to form transient membrane channels allows for the transport of arginine-rich peptides into the cell interior, affecting cellular activities . Furthermore, this compound has been observed to enhance the cellular uptake of biomolecules, potentially altering cellular functions and processes .
Molecular Mechanism
The molecular mechanism of this compound involves its interaction with guanidinium groups and cell membrane components. The compound facilitates the translocation of arginine-rich molecules across the plasma membrane in an energy-independent manner . This process involves the interaction of deprotonated fatty acids with guanidinium groups, leading to the formation of transient membrane channels that allow the transport of peptides into the cell interior . Once inside the cell, the peptides are released, and the membrane channels reseal, completing the translocation process .
Temporal Effects in Laboratory Settings
In laboratory settings, the effects of this compound can change over time. The compound’s stability and degradation are influenced by various factors, including temperature and the presence of other chemicals . Studies have shown that this compound can maintain its properties over extended periods, making it suitable for long-term experiments . Its effects on cellular function may vary depending on the duration of exposure and the specific experimental conditions .
Dosage Effects in Animal Models
The effects of this compound vary with different dosages in animal models. At lower doses, the compound may facilitate the uptake of bioactive molecules without causing significant adverse effects . At higher doses, this compound may exhibit toxic or adverse effects, impacting cellular functions and overall health . It is essential to determine the appropriate dosage to achieve the desired effects while minimizing potential toxicity .
Metabolic Pathways
This compound is involved in various metabolic pathways. It interacts with enzymes and cofactors that play crucial roles in metabolic processes . The compound’s guanidinium groups are essential for its participation in metabolic reactions, influencing metabolic flux and metabolite levels . Additionally, this compound has been shown to affect the stability and functionality of metabolic enzymes, further impacting metabolic pathways .
Transport and Distribution
The transport and distribution of this compound within cells and tissues are influenced by its interactions with transporters and binding proteins . The compound’s guanidinium groups facilitate its uptake and distribution within cells, allowing it to reach specific cellular compartments . Additionally, this compound can accumulate in certain tissues, affecting its overall distribution and localization .
Subcellular Localization
This compound exhibits specific subcellular localization patterns. It can be directed to various cellular compartments, including the cytoplasm, nucleus, and mitochondria . The compound’s targeting signals and post-translational modifications play crucial roles in its localization, influencing its activity and function within different subcellular regions . Understanding the subcellular localization of this compound is essential for elucidating its biochemical and cellular effects.
Preparation Methods
Synthetic Routes and Reaction Conditions: Hexamethylguanidinium chloride can be synthesized by reacting tetramethylguanidine with an alkylating agent in the presence of a suitable catalyst . The reaction typically involves the following steps:
Formation of Tetramethylguanidine: Tetramethylguanidine is prepared by reacting cyanamide with methylamine.
Alkylation: Tetramethylguanidine is then alkylated using methyl iodide or methyl chloride to form this compound.
Industrial Production Methods: In industrial settings, the production of this compound involves similar synthetic routes but on a larger scale. The reaction conditions are optimized to ensure high yield and purity. The use of continuous flow reactors and advanced purification techniques, such as recrystallization and chromatography, are common in industrial production.
Chemical Reactions Analysis
Types of Reactions: Hexamethylguanidinium chloride undergoes various chemical reactions, including:
Nucleophilic Substitution: It can participate in nucleophilic substitution reactions where the chloride ion is replaced by other nucleophiles.
Oxidation and Reduction: The compound can undergo oxidation and reduction reactions, although these are less common.
Common Reagents and Conditions:
Nucleophilic Substitution: Common reagents include sodium hydroxide, potassium hydroxide, and other strong nucleophiles. The reactions are typically carried out in polar solvents like water or alcohol.
Oxidation and Reduction: Oxidizing agents such as hydrogen peroxide and reducing agents like sodium borohydride can be used under controlled conditions.
Major Products: The major products formed from these reactions depend on the specific reagents and conditions used. For example, nucleophilic substitution with sodium hydroxide can yield hexamethylguanidine and sodium chloride.
Scientific Research Applications
Hexamethylguanidinium chloride has a wide range of applications in scientific research:
Chemistry: It is used as a catalyst in organic synthesis, particularly in the preparation of alkyl thiochloroformates.
Medicine: Research is ongoing to explore its potential use in drug delivery systems and as a stabilizing agent for certain pharmaceuticals.
Comparison with Similar Compounds
- Pentamethylguanidinium chloride
- Tetramethylguanidinium chloride
- Guanidinium chloride
Properties
IUPAC Name |
bis(dimethylamino)methylidene-dimethylazanium;chloride | |
---|---|---|
Source | PubChem | |
URL | https://pubchem.ncbi.nlm.nih.gov | |
Description | Data deposited in or computed by PubChem | |
InChI |
InChI=1S/C7H18N3.ClH/c1-8(2)7(9(3)4)10(5)6;/h1-6H3;1H/q+1;/p-1 | |
Source | PubChem | |
URL | https://pubchem.ncbi.nlm.nih.gov | |
Description | Data deposited in or computed by PubChem | |
InChI Key |
WKHWMSFGRUVCNP-UHFFFAOYSA-M | |
Source | PubChem | |
URL | https://pubchem.ncbi.nlm.nih.gov | |
Description | Data deposited in or computed by PubChem | |
Canonical SMILES |
CN(C)C(=[N+](C)C)N(C)C.[Cl-] | |
Source | PubChem | |
URL | https://pubchem.ncbi.nlm.nih.gov | |
Description | Data deposited in or computed by PubChem | |
Molecular Formula |
C7H18ClN3 | |
Source | PubChem | |
URL | https://pubchem.ncbi.nlm.nih.gov | |
Description | Data deposited in or computed by PubChem | |
DSSTOX Substance ID |
DTXSID20374715 | |
Record name | Hexamethylguanidinium chloride | |
Source | EPA DSSTox | |
URL | https://comptox.epa.gov/dashboard/DTXSID20374715 | |
Description | DSSTox provides a high quality public chemistry resource for supporting improved predictive toxicology. | |
Molecular Weight |
179.69 g/mol | |
Source | PubChem | |
URL | https://pubchem.ncbi.nlm.nih.gov | |
Description | Data deposited in or computed by PubChem | |
CAS No. |
30388-20-6 | |
Record name | Hexamethylguanidinium chloride | |
Source | EPA DSSTox | |
URL | https://comptox.epa.gov/dashboard/DTXSID20374715 | |
Description | DSSTox provides a high quality public chemistry resource for supporting improved predictive toxicology. | |
Retrosynthesis Analysis
AI-Powered Synthesis Planning: Our tool employs the Template_relevance Pistachio, Template_relevance Bkms_metabolic, Template_relevance Pistachio_ringbreaker, Template_relevance Reaxys, Template_relevance Reaxys_biocatalysis model, leveraging a vast database of chemical reactions to predict feasible synthetic routes.
One-Step Synthesis Focus: Specifically designed for one-step synthesis, it provides concise and direct routes for your target compounds, streamlining the synthesis process.
Accurate Predictions: Utilizing the extensive PISTACHIO, BKMS_METABOLIC, PISTACHIO_RINGBREAKER, REAXYS, REAXYS_BIOCATALYSIS database, our tool offers high-accuracy predictions, reflecting the latest in chemical research and data.
Strategy Settings
Precursor scoring | Relevance Heuristic |
---|---|
Min. plausibility | 0.01 |
Model | Template_relevance |
Template Set | Pistachio/Bkms_metabolic/Pistachio_ringbreaker/Reaxys/Reaxys_biocatalysis |
Top-N result to add to graph | 6 |
Feasible Synthetic Routes
Q1: What is the structure of Hexamethylguanidinium chloride and what are its key physicochemical properties?
A1: this compound is an organic salt with the molecular formula C7H18N3Cl. It has a molecular weight of 179.71 g/mol. [] The structure consists of a central carbon atom bonded to three dimethylamino groups (N(CH3)2), resulting in a positively charged guanidinium ion. This cation is balanced by a chloride anion. While spectroscopic data isn't extensively discussed in the provided papers, the presence of C-N double bond character within the guanidinium ion is highlighted, suggesting charge delocalization across the NCN planes. []
Q2: How is this compound synthesized?
A2: There are multiple synthetic routes to this compound:
- From Tetramethylchloroformamidinium chloride: Reacting Tetramethylchloroformamidinium chloride (C5H12Cl2N2) with dimethylamine (CH3)2NH generates this compound in situ. []
- From N,N,N′,N′-Tetramethylurea: Treating a mixture of N,N,N′,N′-Tetramethylurea ((CH3)2N)2CO and phthaloyl chloride (C8H4Cl2O2) with dimethyltrimethylsilylamine (C5H15NSi) yields this compound. Tetramethylchloroformamidinium chloride is an intermediate in this reaction. []
- From this compound and Alkoxides: Reacting this compound with sodium alkoxides produces Alkoxy-N,N,N′,N′,N″,N″-hexamethylmethanetriamines. []
- Reduction of this compound: Reduction of this compound with a mixture of sodium hydride (NaH) and either sodium bis(2-methoxyethoxy)aluminum hydride (NaAlH2(OCH2CH2OCH3)2) or trimethyl borate B(OCH3)3 also yields this compound. []
Q3: What are the applications of this compound in organic synthesis?
A3: this compound plays a crucial role as a reagent in various organic reactions:
- Synthesis of Orthoamide Derivatives: It acts as a precursor for the synthesis of various orthoamide derivatives, including those derived from ethynylated terpenes. []
- Preparation of Vinylogous Guanidinium Salts: It reacts with cyclopropylacetylene to form an orthoamide derivative, which can be further transformed into vinylogous guanidinium salts by treatment with iodine (I2). []
- Synthesis of Ketene Aminals: It facilitates the formation of ketene aminals from weakly acidic CH compounds. []
Q4: Is this compound used in the development of ionic liquids?
A4: Yes, this compound has been explored as a cationic component in the development of Polymerized Ionic Liquids (PILs). [] These PILs are being investigated as potential candidates for anion exchange membranes in alkaline fuel cells.
Q5: Are there any stability concerns associated with this compound?
A5: this compound requires careful handling and storage. It is sensitive to moisture and should be handled under inert conditions with the exclusion of air and water. [] The compound's long-term stability and potential degradation pathways have not been extensively discussed in the provided research.
Q6: What are the potential applications of this compound beyond organic synthesis?
A6: While the provided research primarily focuses on the synthetic utility of this compound, its use as a building block for ionic liquids suggests potential applications in areas such as:
Disclaimer and Information on In-Vitro Research Products
Please be aware that all articles and product information presented on BenchChem are intended solely for informational purposes. The products available for purchase on BenchChem are specifically designed for in-vitro studies, which are conducted outside of living organisms. In-vitro studies, derived from the Latin term "in glass," involve experiments performed in controlled laboratory settings using cells or tissues. It is important to note that these products are not categorized as medicines or drugs, and they have not received approval from the FDA for the prevention, treatment, or cure of any medical condition, ailment, or disease. We must emphasize that any form of bodily introduction of these products into humans or animals is strictly prohibited by law. It is essential to adhere to these guidelines to ensure compliance with legal and ethical standards in research and experimentation.