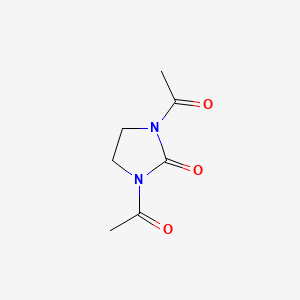
1,3-Diacetyl-2-imidazolidinone
Overview
Description
1,3-Diacetyl-2-imidazolidinone is a chemical compound with the molecular formula C7H10N2O3 . It has a molecular weight of 170.17 g/mol . The IUPAC name for this compound is 1,3-diacetylimidazolidin-2-one .
Synthesis Analysis
The synthesis of imidazolidinones, including this compound, has been explored in various studies . One common approach involves the direct incorporation of the carbonyl group into 1,2-diamines . Another method involves the diamination of olefins . There are also methods that involve the intramolecular hydroamination of linear urea derivatives and aziridine ring expansion .
Molecular Structure Analysis
The molecular structure of this compound consists of 12 heavy atoms . The compound has a complexity of 225 . The InChI string representation of the molecule is InChI=1S/C7H10N2O3/c1-5(10)8-3-4-9(6(2)11)7(8)12/h3-4H2,1-2H3 .
Chemical Reactions Analysis
The reaction mechanism of synthesizing this compound involves a nucleophilic cyclization reaction implemented by two ammonia removal steps . This reaction mechanism has been investigated using first-principles density functional theory .
Physical And Chemical Properties Analysis
This compound has a density of 1.3±0.1 g/cm3 . It has a boiling point of 261.3±23.0 °C at 760 mmHg . The compound has a vapour pressure of 0.0±0.5 mmHg at 25°C . The enthalpy of vaporization is 49.9±3.0 kJ/mol . The compound has a flash point of 112.1±15.0 °C .
Scientific Research Applications
Enantiodivergence and Chiral Auxiliaries
- Chiral Synthons for 1,2-Diamines : Researchers have explored the enantioselective monodeacylation of meso-1,3-diacetyl-2-imidazolidinones, which can produce 1-acetyl-2-imidazolidinones with high enantiomeric excess. These compounds are useful as precursors for efficient chiral auxiliaries (Yokoyama et al., 1998). A similar study demonstrated the use of these compounds in the dissymmetrization of meso-2-imidazolidinones, providing a synthetic route to either enantiomer of 4,5-dimethoxy-2-imidazolidinone derivatives, which are valuable chiral synthons for threo-1,2-diamines (Ishizuka et al., 2004).
Chemical Reactions and Properties
- Metal Ion Reactivity : The reactivity of 1,3-diacetyl-2-imidazolidinone derivatives with butylamine in the presence of various metal ions was studied. Certain bivalent ions like Mg2+, Ca2+, and Mn2+ were found to enhance the electrophilic reactivity of the methoxycarbonyl group in these compounds (Matsumura et al., 1977).
- Thermal Behavior : Research on the thermal behavior and mass spectrum of N,N-di(1-hydroxyalkyl)-2-imidazolidinones, derivatives of this compound, revealed insights into their stability and decomposition process (Shimasaki et al., 1989).
Synthetic Applications
- Enantioselective Organocatalysis : A study demonstrated the first enantioselective organocatalytic amine conjugate addition using imidazolidinone catalysts. This process is significant for the synthesis of beta-amino acids and 1,3-amino alcohol derivatives (Chen et al., 2006).
- Aromatic Finkelstein Iodination : The role of 1,3-dimethyl-2-imidazolidinone as a solvent in the aromatic Finkelstein iodination reaction was highlighted in a study, showcasing its effectiveness in converting aryl bromides to aryl iodides (Yamashita et al., 2012).
Molecular Studies
- Hydrogen Bonding and Resonance : Research on the Raman spectra of 1,3-dimethyl-2-imidazolidinone suggested molecular deformation due to hydrogen bonding to its nitrogen atoms, particularly at low temperatures (Fukushima & Haraoka, 1985).
Safety and Hazards
1,3-Diacetyl-2-imidazolidinone is harmful if swallowed, in contact with skin, or if inhaled . Precautionary measures include avoiding breathing dust, washing skin thoroughly after handling, not eating, drinking, or smoking when using this product, and using only outdoors or in a well-ventilated area .
Future Directions
Mechanism of Action
Target of Action
It’s known that imidazolidin-2-ones and their analogues are omnipresent structural motifs of pharmaceuticals, natural products, chiral auxiliaries, and intermediates in organic syntheses .
Mode of Action
It’s known that the formation of imidazolidin-2-ones from 1,2-diamines and co2 can be efficiently achieved using certain promoters and activators .
Biochemical Pathways
It’s known that imidazolidin-2-ones can be synthesized through various routes, including the direct incorporation of the carbonyl group into 1,2-diamines, the diamination of olefins, the intramolecular hydroamination of linear urea derivatives, and aziridine ring expansion .
Pharmacokinetics
It’s known that the compound has excellent thermal and chemical stability, as well as low viscosity and high dielectric constant, which makes it an excellent electrolyte solvent .
Result of Action
It’s known that imidazolidin-2-ones and their analogues can be transformed into a broad variety of complex structures .
Action Environment
Environmental factors can influence the action, efficacy, and stability of 1,3-Diacetyl-2-imidazolidinone. For instance, it’s known that the compound is stable even in the presence of acids and alkalis . Moreover, it’s been found that water can serve as a proton exchange bridge, facilitating proton migration by shortening the migration distance, which can significantly improve the kinetics of certain reactions .
properties
IUPAC Name |
1,3-diacetylimidazolidin-2-one | |
---|---|---|
Source | PubChem | |
URL | https://pubchem.ncbi.nlm.nih.gov | |
Description | Data deposited in or computed by PubChem | |
InChI |
InChI=1S/C7H10N2O3/c1-5(10)8-3-4-9(6(2)11)7(8)12/h3-4H2,1-2H3 | |
Source | PubChem | |
URL | https://pubchem.ncbi.nlm.nih.gov | |
Description | Data deposited in or computed by PubChem | |
InChI Key |
PAFPWEIHSLHEJT-UHFFFAOYSA-N | |
Source | PubChem | |
URL | https://pubchem.ncbi.nlm.nih.gov | |
Description | Data deposited in or computed by PubChem | |
Canonical SMILES |
CC(=O)N1CCN(C1=O)C(=O)C | |
Source | PubChem | |
URL | https://pubchem.ncbi.nlm.nih.gov | |
Description | Data deposited in or computed by PubChem | |
Molecular Formula |
C7H10N2O3 | |
Source | PubChem | |
URL | https://pubchem.ncbi.nlm.nih.gov | |
Description | Data deposited in or computed by PubChem | |
DSSTOX Substance ID |
DTXSID30337073 | |
Record name | 1,3-Diacetyl-2-imidazolidinone | |
Source | EPA DSSTox | |
URL | https://comptox.epa.gov/dashboard/DTXSID30337073 | |
Description | DSSTox provides a high quality public chemistry resource for supporting improved predictive toxicology. | |
Molecular Weight |
170.17 g/mol | |
Source | PubChem | |
URL | https://pubchem.ncbi.nlm.nih.gov | |
Description | Data deposited in or computed by PubChem | |
CAS RN |
5391-40-2 | |
Record name | 1,3-Diacetyl-2-imidazolidinone | |
Source | CAS Common Chemistry | |
URL | https://commonchemistry.cas.org/detail?cas_rn=5391-40-2 | |
Description | CAS Common Chemistry is an open community resource for accessing chemical information. Nearly 500,000 chemical substances from CAS REGISTRY cover areas of community interest, including common and frequently regulated chemicals, and those relevant to high school and undergraduate chemistry classes. This chemical information, curated by our expert scientists, is provided in alignment with our mission as a division of the American Chemical Society. | |
Explanation | The data from CAS Common Chemistry is provided under a CC-BY-NC 4.0 license, unless otherwise stated. | |
Record name | 1,3-Diacetyl-2-imidazolidinone | |
Source | ChemIDplus | |
URL | https://pubchem.ncbi.nlm.nih.gov/substance/?source=chemidplus&sourceid=0005391402 | |
Description | ChemIDplus is a free, web search system that provides access to the structure and nomenclature authority files used for the identification of chemical substances cited in National Library of Medicine (NLM) databases, including the TOXNET system. | |
Record name | 1,3-Diacetyl-2-imidazolidinone | |
Source | EPA DSSTox | |
URL | https://comptox.epa.gov/dashboard/DTXSID30337073 | |
Description | DSSTox provides a high quality public chemistry resource for supporting improved predictive toxicology. | |
Record name | 1,3-Diacetyl-2-imidazolidinone | |
Source | FDA Global Substance Registration System (GSRS) | |
URL | https://gsrs.ncats.nih.gov/ginas/app/beta/substances/899C7KD45F | |
Description | The FDA Global Substance Registration System (GSRS) enables the efficient and accurate exchange of information on what substances are in regulated products. Instead of relying on names, which vary across regulatory domains, countries, and regions, the GSRS knowledge base makes it possible for substances to be defined by standardized, scientific descriptions. | |
Explanation | Unless otherwise noted, the contents of the FDA website (www.fda.gov), both text and graphics, are not copyrighted. They are in the public domain and may be republished, reprinted and otherwise used freely by anyone without the need to obtain permission from FDA. Credit to the U.S. Food and Drug Administration as the source is appreciated but not required. | |
Retrosynthesis Analysis
AI-Powered Synthesis Planning: Our tool employs the Template_relevance Pistachio, Template_relevance Bkms_metabolic, Template_relevance Pistachio_ringbreaker, Template_relevance Reaxys, Template_relevance Reaxys_biocatalysis model, leveraging a vast database of chemical reactions to predict feasible synthetic routes.
One-Step Synthesis Focus: Specifically designed for one-step synthesis, it provides concise and direct routes for your target compounds, streamlining the synthesis process.
Accurate Predictions: Utilizing the extensive PISTACHIO, BKMS_METABOLIC, PISTACHIO_RINGBREAKER, REAXYS, REAXYS_BIOCATALYSIS database, our tool offers high-accuracy predictions, reflecting the latest in chemical research and data.
Strategy Settings
Precursor scoring | Relevance Heuristic |
---|---|
Min. plausibility | 0.01 |
Model | Template_relevance |
Template Set | Pistachio/Bkms_metabolic/Pistachio_ringbreaker/Reaxys/Reaxys_biocatalysis |
Top-N result to add to graph | 6 |
Feasible Synthetic Routes
Disclaimer and Information on In-Vitro Research Products
Please be aware that all articles and product information presented on BenchChem are intended solely for informational purposes. The products available for purchase on BenchChem are specifically designed for in-vitro studies, which are conducted outside of living organisms. In-vitro studies, derived from the Latin term "in glass," involve experiments performed in controlled laboratory settings using cells or tissues. It is important to note that these products are not categorized as medicines or drugs, and they have not received approval from the FDA for the prevention, treatment, or cure of any medical condition, ailment, or disease. We must emphasize that any form of bodily introduction of these products into humans or animals is strictly prohibited by law. It is essential to adhere to these guidelines to ensure compliance with legal and ethical standards in research and experimentation.