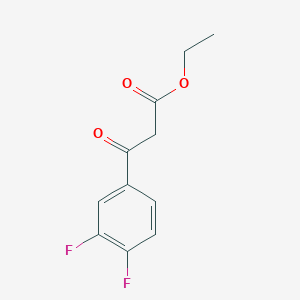
Ethyl 3-(3,4-difluorophenyl)-3-oxopropanoate
Overview
Description
Ethyl 3-(3,4-difluorophenyl)-3-oxopropanoate is a fluorinated β-keto ester characterized by a 3,4-difluorophenyl substituent at the β-position of the propanoate backbone. This compound serves as a critical intermediate in medicinal chemistry, particularly in the synthesis of bioactive molecules targeting receptors such as melanin-concentrating hormone receptor 1 (MCHR1) . Its structural framework allows for versatile modifications, enabling the development of derivatives with tailored pharmacokinetic and pharmacodynamic properties. The electronegative fluorine atoms enhance metabolic stability and influence electronic properties, making it advantageous in drug discovery .
Preparation Methods
Synthetic Routes for Preparation
The preparation of Ethyl 3-(3,4-difluorophenyl)-3-oxopropanoate generally involves the condensation of 3,4-difluorobenzene derivatives with ethyl acetoacetate or related β-ketoester precursors. The following detailed methods have been reported:
Direct Esterification and Acylation Approach
- Starting Materials: 3,4-difluorobenzoyl chloride or 3,4-difluorobenzoic acid derivatives.
- Key Reaction: Claisen condensation or acylation of ethyl acetate derivatives with 3,4-difluorophenyl precursors.
- Typical Conditions: Use of base catalysts such as sodium ethoxide or potassium tert-butoxide in ethanol or ethyl acetate solvent systems.
- Outcome: Formation of the β-ketoester with the desired difluorophenyl substitution.
Malonate Alkylation Route
- Starting Materials: Ethyl potassium malonate and 3,4-difluorobenzyl halides.
- Reaction: Nucleophilic substitution where the malonate anion displaces the halide on the difluorobenzyl moiety.
- Subsequent Step: Hydrolysis and decarboxylation under acidic or basic conditions to yield the β-ketoester.
- Advantages: This method allows for selective introduction of the difluorophenyl group and control over substitution patterns.
Enol Ether Intermediate Cyclization
- Intermediate Formation: Reflux of 2,4,5-trifluoro-β-oxobenzenepropanoic acid ethyl ester with triethylorthoformate and acetic anhydride to form an enol ether intermediate.
- Substitution: The enol ether is then reacted with amines or substituted phenyl groups to introduce the difluorophenyl moiety.
- Cyclization: Treatment with bases such as sodium hydride or lithium hydroxide induces ring closure or ester hydrolysis to finalize the β-ketoester structure.
- Utility: This method is useful for synthesizing derivatives with additional substitutions on the aromatic ring.
Reaction Conditions and Techniques
- Solvents: Common solvents include ethanol, ethyl acetate, tetrahydrofuran (THF), and dimethyl sulfoxide (DMSO) for dissolution and reaction medium.
- Catalysts and Bases: Sodium ethoxide, potassium tert-butoxide, lithium hydroxide, and sodium hydride are frequently employed to facilitate condensation, substitution, and hydrolysis steps.
- Temperature: Reactions are typically conducted under reflux conditions (60–110 °C) to promote completion.
- Physical Methods: Vortexing, ultrasound, and hot water baths are used to aid dissolution and reaction kinetics during preparation of stock solutions and intermediates.
Analytical Data and Yield Information
Parameter | Details |
---|---|
Molecular Weight | Approx. 224.2 g/mol (C12H11F2O3) |
Purity Assessment | Confirmed by NMR, HPLC, and mass spectrometry |
Typical Yields | 70–85% depending on method and scale |
Physical State | Colorless to pale yellow liquid or solid |
Solubility | Soluble in DMSO, ethanol, and acetone |
Stock Solution Preparation (For Experimental Use)
Based on data from GlpBio, preparation of stock solutions of this compound involves precise calculations for molarity and volume, as shown below:
Amount of Compound (mg) | Volume for 1 mM (mL) | Volume for 5 mM (mL) | Volume for 10 mM (mL) |
---|---|---|---|
1 | 4.3823 | 0.8765 | 0.4382 |
5 | 21.9114 | 4.3823 | 2.1911 |
10 | 43.8227 | 8.7645 | 4.3823 |
Note: Physical methods such as vortexing, ultrasound, or hot water baths are recommended to ensure complete dissolution before use in further reactions or biological assays.
Research Findings and Optimization Notes
- Purity and Yield Optimization: Reaction parameters such as temperature, solvent choice, and base concentration significantly influence the yield and purity of the final product.
- Substitution Effects: The presence of fluorine atoms at the 3,4-positions on the phenyl ring enhances the electrophilicity of the carbonyl carbon, facilitating nucleophilic attack during condensation.
- Stability: The compound exhibits good stability under standard laboratory storage conditions but requires protection from moisture to avoid hydrolysis of the ester group.
- Scale-Up Potential: The synthetic routes described are amenable to scale-up with appropriate control of reaction parameters and purification steps.
Summary Table of Preparation Methods
Method | Starting Materials | Key Reagents/Bases | Conditions | Advantages | Disadvantages |
---|---|---|---|---|---|
Direct Esterification | 3,4-difluorobenzoyl chloride, ethyl acetate | Sodium ethoxide, ethanol | Reflux, 60–80 °C | Simple, straightforward | Moderate yields, side reactions |
Malonate Alkylation | Ethyl potassium malonate, difluorobenzyl halide | Potassium carbonate, solvents | Room temp to reflux | Selective substitution | Multi-step, requires purification |
Enol Ether Intermediate Cyclization | 2,4,5-trifluoro-β-oxobenzenepropanoic acid ethyl ester | Triethylorthoformate, acetic anhydride, sodium hydride | Reflux, base treatment | Allows diverse substitutions | More complex, longer synthesis |
Chemical Reactions Analysis
Types of Reactions
Ethyl 3-(3,4-difluorophenyl)-3-oxopropanoate undergoes various chemical reactions, including:
Oxidation: The compound can be oxidized to form corresponding carboxylic acids.
Reduction: Reduction reactions can convert the ester group to an alcohol.
Substitution: The fluorine atoms on the phenyl ring can be substituted with other functional groups through nucleophilic aromatic substitution reactions.
Common Reagents and Conditions
Oxidation: Common oxidizing agents include potassium permanganate (KMnO4) and chromium trioxide (CrO3).
Reduction: Reducing agents such as lithium aluminum hydride (LiAlH4) or sodium borohydride (NaBH4) are used.
Substitution: Nucleophiles such as amines or thiols can be used for substitution reactions under basic conditions.
Major Products
Oxidation: 3-(3,4-difluorophenyl)-3-oxopropanoic acid.
Reduction: Ethyl 3-(3,4-difluorophenyl)-3-hydroxypropanoate.
Substitution: Various substituted phenylpropanoates depending on the nucleophile used.
Scientific Research Applications
Ethyl 3-(3,4-difluorophenyl)-3-oxopropanoate has several applications in scientific research:
Chemistry: It is used as a building block in the synthesis of more complex organic molecules.
Biology: The compound is studied for its potential biological activities, including antimicrobial and anti-inflammatory properties.
Medicine: Research is ongoing to explore its potential as a pharmaceutical intermediate.
Industry: It is used in the production of specialty chemicals and materials.
Mechanism of Action
The mechanism of action of Ethyl 3-(3,4-difluorophenyl)-3-oxopropanoate involves its interaction with specific molecular targets. The ester group can undergo hydrolysis to release the active carboxylic acid, which can interact with enzymes or receptors in biological systems. The fluorine atoms enhance the compound’s stability and bioavailability by influencing its electronic properties and metabolic pathways.
Comparison with Similar Compounds
Comparative Analysis with Structural Analogs
Substituent Effects: Fluorine vs. Chlorine
Fluorinated and chlorinated analogs of β-keto esters are widely studied for their distinct electronic and steric profiles:
*Similarity scores (0–1 scale) based on structural alignment with the target compound .
†Calculated based on molecular formula C₁₁H₁₀F₂O₃.
Key Observations :
- Electronic Effects : Fluorine’s high electronegativity reduces electron density at the aryl ring, enhancing resistance to oxidative metabolism compared to chlorinated analogs .
- Lipophilicity : Fluorine substitution increases logP values marginally compared to chlorine, improving membrane permeability .
- Synthetic Utility : Chlorinated analogs (e.g., 3,4-dichlorophenyl) are more reactive in nucleophilic substitutions due to weaker C–Cl bonds, whereas fluorinated derivatives require harsher conditions .
Positional Isomerism in Fluorinated Derivatives
The position of fluorine substituents significantly impacts physicochemical and biological properties:
‡Solubility measured in dimethyl sulfoxide (DMSO) at 25°C.
*IC₅₀ values for MCHR1 antagonism.
Key Observations :
- Steric Effects : Ortho-substituted derivatives (2-fluoro) exhibit lower solubility due to steric hindrance, whereas para-substituted analogs (4-fluoro) show improved solubility .
- Bioactivity: The 3,4-difluoro derivative demonstrates superior receptor affinity (IC₅₀ = 85 nM) compared to mono-fluoro analogs, likely due to optimized halogen bonding with target proteins .
Comparison with Heterocyclic and Alkylated Derivatives
Ethyl 3-cyclopropyl-3-oxopropanoates and pyridinyl analogs highlight the role of non-aryl substituents:
Key Observations :
- Reactivity : Nitropyridinyl derivatives require milder bases (e.g., DIPEA) compared to fluorobenzyl analogs, which utilize LiCl as a catalyst .
- Biological Targets : Heterocyclic substituents (e.g., pyridinyl) broaden applications in anticancer research, while alkylated derivatives are explored for antimicrobial activity .
Biological Activity
Ethyl 3-(3,4-difluorophenyl)-3-oxopropanoate is a compound of significant interest in medicinal chemistry due to its potential biological activities. This article reviews its biological effects, mechanisms of action, and relevant research findings.
Chemical Structure and Properties
This compound has the molecular formula and a molecular weight of approximately 228.2 g/mol. The compound features a fluorinated phenyl group and a keto ester functional group, contributing to its reactivity and biological interactions. The presence of fluorine atoms enhances the compound's stability and bioavailability by influencing electronic properties and metabolic pathways.
The biological activity of this compound is attributed to its interaction with specific molecular targets, including enzymes and receptors involved in various metabolic pathways. The ester group can undergo hydrolysis to release the active carboxylic acid form, which can modulate enzyme activity or receptor binding . This mechanism suggests potential applications in therapeutic interventions.
Biological Activities
Research has indicated that this compound exhibits several biological activities:
- Antimicrobial Properties : Preliminary studies suggest that this compound may possess antimicrobial effects, although specific mechanisms remain to be fully elucidated.
- Anti-inflammatory Effects : The compound has been investigated for its potential to reduce inflammation through modulation of pro-inflammatory cytokines.
- Anticancer Potential : Some derivatives of this compound have been explored for their efficacy as anticancer agents, showing promising results in inhibiting tumor cell proliferation in vitro and in vivo .
Table 1: Summary of Biological Activities
Activity Type | Observations | References |
---|---|---|
Antimicrobial | Exhibits activity against various pathogens | , |
Anti-inflammatory | Reduces pro-inflammatory cytokines | , |
Anticancer | Inhibits cancer cell proliferation | , |
Case Study: Anticancer Activity
A study published in the journal "Cancer Research" evaluated the anticancer effects of this compound on human colon cancer cells. The compound was found to inhibit cell growth with an IC50 value significantly lower than that of standard chemotherapeutics such as etoposide. In xenograft models, treatment with the compound resulted in reduced tumor size compared to controls .
Future Directions
The ongoing research into this compound highlights its potential as a lead compound for drug development. Further studies are needed to elucidate its precise mechanisms of action, optimize its pharmacological properties, and evaluate its safety profile in clinical settings.
Q & A
Basic Research Questions
Q. What are the primary synthetic routes for Ethyl 3-(3,4-difluorophenyl)-3-oxopropanoate, and how can reaction yields be optimized?
The compound is commonly synthesized via condensation of dilithiomonoethylmalonate with fluorinated benzoyl chlorides under cryogenic conditions (e.g., −78°C), followed by purification via flash chromatography . Optimizing yields involves controlling reaction stoichiometry, using anhydrous solvents, and selecting catalysts (e.g., CaSO₄ or molecular sieves) to absorb byproducts like water . For example, refluxing with ammonium formate in ethanol under N₂ for 8–144 hours achieves yields >50% in pyrimidinone syntheses .
Q. How can researchers characterize the purity and structural identity of this compound?
Key methods include:
- NMR spectroscopy : ¹H/¹³C NMR to confirm the β-ketoester backbone and fluorophenyl substituents (e.g., δ ~4.25 ppm for the ethyl ester group and aromatic proton shifts at δ 7.3–7.8 ppm) .
- Mass spectrometry : Exact mass analysis (e.g., molecular weight 214.046 g/mol for methyl analogs) to verify molecular formula .
- Chromatography : HPLC or TLC to assess purity, with petroleum ether:ethyl acetate (20:1) as a common solvent system .
Q. What safety precautions are necessary when handling this compound?
While not classified as hazardous under GHS, standard lab practices include:
- Using PPE (gloves, goggles) to avoid skin/eye contact.
- Working in a fume hood due to potential irritancy of fluorinated intermediates.
- Storing under inert gas (N₂/Ar) to prevent hydrolysis of the β-ketoester group .
Advanced Research Questions
Q. How do structural modifications (e.g., fluorination patterns) influence reactivity in heterocyclic synthesis?
The 3,4-difluorophenyl group enhances electrophilicity at the keto position, facilitating nucleophilic attacks (e.g., by guanidine or amines) to form pyrimidinones or acrylates . Comparative studies show that 2-fluorophenyl analogs exhibit lower reactivity due to steric hindrance, while 4-fluorophenyl derivatives undergo faster condensations . For example, ethyl 3-(4-fluorophenyl)-3-oxopropanoate reacts with 2,3-dimethoxybenzenamine 30% faster than its 2-fluoro analog .
Q. What strategies resolve contradictions in reported synthetic yields for derivatives of this compound?
Discrepancies often arise from:
- Catalyst selection : Molecular sieves vs. CaSO₄, where the latter may absorb excess water more effectively in ethanol reflux .
- Reaction duration : Extended reflux (144 hours vs. 8 hours) improves yields in sluggish condensations .
- Purification methods : Flash chromatography with gradient elution resolves co-eluting byproducts, which are common in fluorinated systems .
Q. How can computational modeling guide the design of novel derivatives for biological screening?
DFT calculations predict electrophilic reactivity at the β-keto position, while molecular docking identifies potential interactions with biological targets (e.g., enzyme active sites). For instance, analogs with electron-withdrawing groups (e.g., −CN or −NO₂) on the phenyl ring show enhanced binding to Mycobacterium tuberculosis Pks13 in silico .
Q. What methodologies assess the compound’s role in radical-mediated reactions?
Cobalt-catalyzed radical reactions can functionalize the β-ketoester backbone. For example, ethyl 3-((3,4-dichlorophenyl)amino)-3-oxopropanoate undergoes radical coupling with alkenes under Co(II) catalysis to form γ-lactams, characterized via ESR to detect radical intermediates .
Q. Methodological Challenges and Solutions
Q. How to address incomplete physicochemical data (e.g., solubility, melting point) for this compound?
- Experimental determination : Use differential scanning calorimetry (DSC) for melting points and shake-flask methods for solubility in DMSO/water mixtures.
- Predictive models : Employ Hansen solubility parameters or COSMO-RS simulations to estimate solubility in untested solvents .
Q. What analytical techniques differentiate between keto-enol tautomers in solution?
Properties
IUPAC Name |
ethyl 3-(3,4-difluorophenyl)-3-oxopropanoate | |
---|---|---|
Source | PubChem | |
URL | https://pubchem.ncbi.nlm.nih.gov | |
Description | Data deposited in or computed by PubChem | |
InChI |
InChI=1S/C11H10F2O3/c1-2-16-11(15)6-10(14)7-3-4-8(12)9(13)5-7/h3-5H,2,6H2,1H3 | |
Source | PubChem | |
URL | https://pubchem.ncbi.nlm.nih.gov | |
Description | Data deposited in or computed by PubChem | |
InChI Key |
IEJMFJYBMANBQM-UHFFFAOYSA-N | |
Source | PubChem | |
URL | https://pubchem.ncbi.nlm.nih.gov | |
Description | Data deposited in or computed by PubChem | |
Canonical SMILES |
CCOC(=O)CC(=O)C1=CC(=C(C=C1)F)F | |
Source | PubChem | |
URL | https://pubchem.ncbi.nlm.nih.gov | |
Description | Data deposited in or computed by PubChem | |
Molecular Formula |
C11H10F2O3 | |
Source | PubChem | |
URL | https://pubchem.ncbi.nlm.nih.gov | |
Description | Data deposited in or computed by PubChem | |
DSSTOX Substance ID |
DTXSID90374282 | |
Record name | ethyl 3-(3,4-difluorophenyl)-3-oxopropanoate | |
Source | EPA DSSTox | |
URL | https://comptox.epa.gov/dashboard/DTXSID90374282 | |
Description | DSSTox provides a high quality public chemistry resource for supporting improved predictive toxicology. | |
Molecular Weight |
228.19 g/mol | |
Source | PubChem | |
URL | https://pubchem.ncbi.nlm.nih.gov | |
Description | Data deposited in or computed by PubChem | |
CAS No. |
252955-07-0 | |
Record name | ethyl 3-(3,4-difluorophenyl)-3-oxopropanoate | |
Source | EPA DSSTox | |
URL | https://comptox.epa.gov/dashboard/DTXSID90374282 | |
Description | DSSTox provides a high quality public chemistry resource for supporting improved predictive toxicology. | |
Record name | 252955-07-0 | |
Source | European Chemicals Agency (ECHA) | |
URL | https://echa.europa.eu/information-on-chemicals | |
Description | The European Chemicals Agency (ECHA) is an agency of the European Union which is the driving force among regulatory authorities in implementing the EU's groundbreaking chemicals legislation for the benefit of human health and the environment as well as for innovation and competitiveness. | |
Explanation | Use of the information, documents and data from the ECHA website is subject to the terms and conditions of this Legal Notice, and subject to other binding limitations provided for under applicable law, the information, documents and data made available on the ECHA website may be reproduced, distributed and/or used, totally or in part, for non-commercial purposes provided that ECHA is acknowledged as the source: "Source: European Chemicals Agency, http://echa.europa.eu/". Such acknowledgement must be included in each copy of the material. ECHA permits and encourages organisations and individuals to create links to the ECHA website under the following cumulative conditions: Links can only be made to webpages that provide a link to the Legal Notice page. | |
Synthesis routes and methods
Procedure details
Retrosynthesis Analysis
AI-Powered Synthesis Planning: Our tool employs the Template_relevance Pistachio, Template_relevance Bkms_metabolic, Template_relevance Pistachio_ringbreaker, Template_relevance Reaxys, Template_relevance Reaxys_biocatalysis model, leveraging a vast database of chemical reactions to predict feasible synthetic routes.
One-Step Synthesis Focus: Specifically designed for one-step synthesis, it provides concise and direct routes for your target compounds, streamlining the synthesis process.
Accurate Predictions: Utilizing the extensive PISTACHIO, BKMS_METABOLIC, PISTACHIO_RINGBREAKER, REAXYS, REAXYS_BIOCATALYSIS database, our tool offers high-accuracy predictions, reflecting the latest in chemical research and data.
Strategy Settings
Precursor scoring | Relevance Heuristic |
---|---|
Min. plausibility | 0.01 |
Model | Template_relevance |
Template Set | Pistachio/Bkms_metabolic/Pistachio_ringbreaker/Reaxys/Reaxys_biocatalysis |
Top-N result to add to graph | 6 |
Feasible Synthetic Routes
Disclaimer and Information on In-Vitro Research Products
Please be aware that all articles and product information presented on BenchChem are intended solely for informational purposes. The products available for purchase on BenchChem are specifically designed for in-vitro studies, which are conducted outside of living organisms. In-vitro studies, derived from the Latin term "in glass," involve experiments performed in controlled laboratory settings using cells or tissues. It is important to note that these products are not categorized as medicines or drugs, and they have not received approval from the FDA for the prevention, treatment, or cure of any medical condition, ailment, or disease. We must emphasize that any form of bodily introduction of these products into humans or animals is strictly prohibited by law. It is essential to adhere to these guidelines to ensure compliance with legal and ethical standards in research and experimentation.