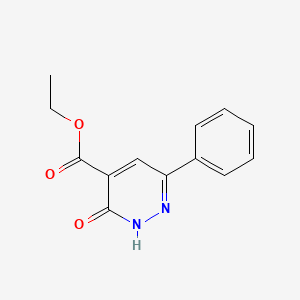
Ethyl 3-oxo-6-phenyl-2,3-dihydropyridazine-4-carboxylate
Overview
Description
Ethyl 3-oxo-6-phenyl-2,3-dihydropyridazine-4-carboxylate is a chemical compound with the molecular formula C13H12N2O3. It is a member of the pyridazine family, characterized by a six-membered ring containing two adjacent nitrogen atoms.
Preparation Methods
Synthetic Routes and Reaction Conditions: The synthesis of ethyl 3-oxo-6-phenyl-2,3-dihydropyridazine-4-carboxylate typically involves the condensation of appropriate starting materials under controlled conditions. One common method involves the reaction of diethyl malonate with a hydrazine derivative, followed by cyclization and esterification steps .
Industrial Production Methods: While specific industrial production methods for this compound are not widely documented, the general approach would involve scaling up the laboratory synthesis procedures. This would include optimizing reaction conditions, such as temperature, pressure, and solvent choice, to ensure high yield and purity .
Chemical Reactions Analysis
Types of Reactions: Ethyl 3-oxo-6-phenyl-2,3-dihydropyridazine-4-carboxylate can undergo various chemical reactions, including:
Oxidation: This reaction can introduce additional functional groups or modify existing ones.
Reduction: This can convert the oxo group to a hydroxyl group or other reduced forms.
Substitution: This involves replacing one functional group with another, often using nucleophilic or electrophilic reagents.
Common Reagents and Conditions:
Oxidation: Common oxidizing agents include potassium permanganate (KMnO4) and chromium trioxide (CrO3).
Reduction: Reducing agents such as sodium borohydride (NaBH4) or lithium aluminum hydride (LiAlH4) are often used.
Substitution: Reagents like halogens (e.g., chlorine, bromine) or organometallic compounds can facilitate substitution reactions.
Major Products Formed: The products of these reactions depend on the specific conditions and reagents used. For example, oxidation might yield carboxylic acids or ketones, while reduction could produce alcohols or amines .
Scientific Research Applications
Ethyl 3-oxo-6-phenyl-2,3-dihydropyridazine-4-carboxylate has several applications in scientific research:
Chemistry: It serves as a building block for synthesizing more complex molecules and studying reaction mechanisms.
Biology: It is used in the development of enzyme inhibitors and other bioactive compounds.
Medicine: Research is ongoing into its potential as a therapeutic agent for various diseases, including diabetes and cancer.
Mechanism of Action
The mechanism of action of ethyl 3-oxo-6-phenyl-2,3-dihydropyridazine-4-carboxylate involves its interaction with specific molecular targets. For instance, it may inhibit certain enzymes by binding to their active sites, thereby blocking substrate access and reducing enzyme activity. This can lead to various biological effects, such as reduced blood glucose levels in the case of diabetes .
Comparison with Similar Compounds
- Ethyl 3-oxo-5,6-diphenyl-2,3-dihydropyridazine-4-carboxylate
- Ethyl 6-methyl-2-oxo-4-(3-(2,4,5-trimethoxyphenyl)-1H-pyrazol-1-yl)-2,3-dihydropyridazine-4-carboxylate
Comparison: Ethyl 3-oxo-6-phenyl-2,3-dihydropyridazine-4-carboxylate is unique due to its specific substitution pattern on the pyridazine ring, which can influence its reactivity and biological activity. Compared to similar compounds, it may exhibit different pharmacokinetic properties, making it more suitable for certain applications .
Properties
IUPAC Name |
ethyl 6-oxo-3-phenyl-1H-pyridazine-5-carboxylate | |
---|---|---|
Source | PubChem | |
URL | https://pubchem.ncbi.nlm.nih.gov | |
Description | Data deposited in or computed by PubChem | |
InChI |
InChI=1S/C13H12N2O3/c1-2-18-13(17)10-8-11(14-15-12(10)16)9-6-4-3-5-7-9/h3-8H,2H2,1H3,(H,15,16) | |
Source | PubChem | |
URL | https://pubchem.ncbi.nlm.nih.gov | |
Description | Data deposited in or computed by PubChem | |
InChI Key |
GNOBFXNVLBLIAZ-UHFFFAOYSA-N | |
Source | PubChem | |
URL | https://pubchem.ncbi.nlm.nih.gov | |
Description | Data deposited in or computed by PubChem | |
Canonical SMILES |
CCOC(=O)C1=CC(=NNC1=O)C2=CC=CC=C2 | |
Source | PubChem | |
URL | https://pubchem.ncbi.nlm.nih.gov | |
Description | Data deposited in or computed by PubChem | |
Molecular Formula |
C13H12N2O3 | |
Source | PubChem | |
URL | https://pubchem.ncbi.nlm.nih.gov | |
Description | Data deposited in or computed by PubChem | |
DSSTOX Substance ID |
DTXSID10363072 | |
Record name | Ethyl 3-oxo-6-phenyl-2,3-dihydropyridazine-4-carboxylate | |
Source | EPA DSSTox | |
URL | https://comptox.epa.gov/dashboard/DTXSID10363072 | |
Description | DSSTox provides a high quality public chemistry resource for supporting improved predictive toxicology. | |
Molecular Weight |
244.25 g/mol | |
Source | PubChem | |
URL | https://pubchem.ncbi.nlm.nih.gov | |
Description | Data deposited in or computed by PubChem | |
CAS No. |
34753-27-0 | |
Record name | Ethyl 3-oxo-6-phenyl-2,3-dihydropyridazine-4-carboxylate | |
Source | EPA DSSTox | |
URL | https://comptox.epa.gov/dashboard/DTXSID10363072 | |
Description | DSSTox provides a high quality public chemistry resource for supporting improved predictive toxicology. | |
Retrosynthesis Analysis
AI-Powered Synthesis Planning: Our tool employs the Template_relevance Pistachio, Template_relevance Bkms_metabolic, Template_relevance Pistachio_ringbreaker, Template_relevance Reaxys, Template_relevance Reaxys_biocatalysis model, leveraging a vast database of chemical reactions to predict feasible synthetic routes.
One-Step Synthesis Focus: Specifically designed for one-step synthesis, it provides concise and direct routes for your target compounds, streamlining the synthesis process.
Accurate Predictions: Utilizing the extensive PISTACHIO, BKMS_METABOLIC, PISTACHIO_RINGBREAKER, REAXYS, REAXYS_BIOCATALYSIS database, our tool offers high-accuracy predictions, reflecting the latest in chemical research and data.
Strategy Settings
Precursor scoring | Relevance Heuristic |
---|---|
Min. plausibility | 0.01 |
Model | Template_relevance |
Template Set | Pistachio/Bkms_metabolic/Pistachio_ringbreaker/Reaxys/Reaxys_biocatalysis |
Top-N result to add to graph | 6 |
Feasible Synthetic Routes
Disclaimer and Information on In-Vitro Research Products
Please be aware that all articles and product information presented on BenchChem are intended solely for informational purposes. The products available for purchase on BenchChem are specifically designed for in-vitro studies, which are conducted outside of living organisms. In-vitro studies, derived from the Latin term "in glass," involve experiments performed in controlled laboratory settings using cells or tissues. It is important to note that these products are not categorized as medicines or drugs, and they have not received approval from the FDA for the prevention, treatment, or cure of any medical condition, ailment, or disease. We must emphasize that any form of bodily introduction of these products into humans or animals is strictly prohibited by law. It is essential to adhere to these guidelines to ensure compliance with legal and ethical standards in research and experimentation.