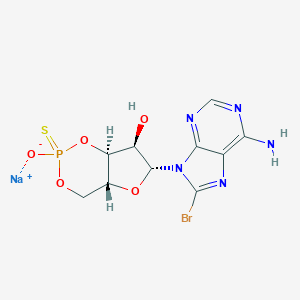
8-Bromoadenosine 3',5'-cyclic monophosphothiaoate, Sp-isomer sodium salt
Overview
Description
8-Bromoadenosine 3’,5’-Cyclic Monophosphothioate Sp-Isomer Sodium Salt is an activator of cAMP-dependent PKA . It is a brominated cyclic mononucleotide .
Physical And Chemical Properties Analysis
8-Bromoadenosine 3’,5’-Cyclic Monophosphothioate Sp-Isomer Sodium Salt is a powder with a molecular weight of 430.08. It is soluble in water (100 mg/mL) and is stored at −20°C .Scientific Research Applications
Promoting Cell Division in Plant Tissue
8-Bromoadenosine 3',5'-cyclic monophosphate, when combined with an auxin, can fully replace the cell-division-promoting activity of cytokinesins or 6-substituted adenylyl cytokinins in excised tobacco pith parenchyma tissue. This derivative is more resistant to degradation by plant phosphodiesterases than adenosine 3',5'-cyclic monophosphate, supporting its role in cell-division regulation (Wood & Braun, 1973).
Stimulating Insulin Release
In isolated pancreatic islets of rats, the Sp-isomer of this compound was a more potent stimulus for insulin release than 8-bromo-cyclic AMP, suggesting its significant role in cAMP-mediated insulin release (Laychock, 1993).
Synthesis and Photochemistry
New derivatives of hydrolysis-resistant 8-bromoadenosine cyclic 3',5'-monophosphate have been synthesized for physiological studies. These compounds serve as efficient intracellular sources of 8-Br-cAMP and 8-Br-cGMP, demonstrating their application in mammalian cell line studies (Hagen et al., 1999).
Attenuating Neutrophil-Mediated Increase in Endothelial Permeability
8-Bromoadenosine 3',5'-cyclic monophosphate was found to significantly attenuate the increase in endothelial permeability after polymorphonuclear leukocytes activation, indicating its potential in preventing endothelial injury (Siflinger‐Birnboim et al., 1993).
Impact on Isotonic Volume Reduction in Jejunal Crypt Epithelial Cells
This compound caused rapid shrinkage to a reduced but stable cell volume in jejunal crypt epithelial cells, showing its role in regulating cell volume via chloride and potassium channels (Macleod et al., 1994).
Enhancing Vasorelaxation via the cAMP Pathway
It was observed that this compound enhanced vasorelaxation to endothelium-independent relaxing agents, indicating its role in the cyclic AMP-dependent pathway in vascular relaxation (Yeung et al., 2006).
Mechanism of Action
Target of Action
The primary target of Rp-8-bromo-Cyclic AMPS (sodium salt), also known as 8-Bromoadenosine 3’,5’-cyclic monophosphothiaoate, Sp-isomer sodium salt or Rp-8-bromo-Cyclic AMPS, is cAMP-dependent protein kinases (PKAs) . PKAs are a group of enzymes that are involved in cellular signal transduction pathways and play a crucial role in regulating various cellular functions.
Mode of Action
Rp-8-bromo-Cyclic AMPS is a cell-permeable cAMP analog . It acts as an antagonist of cAMP-dependent protein kinases (PKAs) and is resistant to hydrolysis by cyclic nucleotide phosphodiesterases . This means it binds to PKAs without activating them, resulting in competitive inhibition .
Biochemical Pathways
The compound’s action primarily affects the cAMP signaling pathway . By antagonizing the activation of PKAs, it modulates the downstream effects of this pathway. The exact downstream effects can vary depending on the specific cellular context, but they often involve changes in gene expression and cellular metabolism.
Pharmacokinetics
While specific ADME (Absorption, Distribution, Metabolism, and Excretion) properties for Rp-8-bromo-Cyclic AMPS are not readily available, it’s known that the compound is a crystalline solid with solubility in DMF (30 mg/ml), DMSO (25 mg/ml), Ethanol (0.5 mg/ml), and PBS (pH 7.2, 10 mg/ml) . These properties suggest that the compound could have reasonable bioavailability, but further studies would be needed to confirm this.
Result of Action
The molecular and cellular effects of Rp-8-bromo-Cyclic AMPS’s action depend on the specific context in which it is used. It’s known that the compound can effectively antagonize camp-dependent activation of pkas . This can lead to a variety of effects, such as changes in gene expression and cellular metabolism.
Action Environment
The action, efficacy, and stability of Rp-8-bromo-Cyclic AMPS can be influenced by various environmental factors. For instance, the pH of the environment can affect the compound’s solubility and therefore its bioavailability . Additionally, the presence of other molecules that can interact with PKAs could potentially influence the compound’s efficacy.
Biochemical Analysis
Biochemical Properties
Rp-8-bromo-Cyclic AMPS (sodium salt) acts as an antagonist of cAMP-dependent protein kinases (PKAs) and is resistant to hydrolysis by cyclic nucleotide phosphodiesterases . It more effectively antagonizes cAMP-dependent activation of purified PKA type I from rabbit muscle than PKA type II from bovine heart .
Cellular Effects
The cellular effects of Rp-8-bromo-Cyclic AMPS (sodium salt) are primarily mediated through its antagonistic action on cAMP-dependent protein kinases. By inhibiting these kinases, it can influence various cellular processes, including cell signaling pathways, gene expression, and cellular metabolism .
Molecular Mechanism
At the molecular level, Rp-8-bromo-Cyclic AMPS (sodium salt) exerts its effects by binding to the active sites of cAMP-dependent protein kinases, thereby preventing their activation . This inhibition can lead to changes in gene expression and other downstream effects .
Temporal Effects in Laboratory Settings
In laboratory settings, Rp-8-bromo-Cyclic AMPS (sodium salt) has been shown to be stable and resistant to degradation . Its effects on cellular function can be observed over extended periods in both in vitro and in vivo studies .
properties
IUPAC Name |
sodium;(4aR,6R,7R,7aS)-6-(6-amino-8-bromopurin-9-yl)-2-oxido-2-sulfanylidene-4a,6,7,7a-tetrahydro-4H-furo[3,2-d][1,3,2]dioxaphosphinin-7-ol | |
---|---|---|
Source | PubChem | |
URL | https://pubchem.ncbi.nlm.nih.gov | |
Description | Data deposited in or computed by PubChem | |
InChI |
InChI=1S/C10H11BrN5O5PS.Na/c11-10-15-4-7(12)13-2-14-8(4)16(10)9-5(17)6-3(20-9)1-19-22(18,23)21-6;/h2-3,5-6,9,17H,1H2,(H,18,23)(H2,12,13,14);/q;+1/p-1/t3-,5-,6-,9-,22?;/m1./s1 | |
Source | PubChem | |
URL | https://pubchem.ncbi.nlm.nih.gov | |
Description | Data deposited in or computed by PubChem | |
InChI Key |
SKJLJCVVXRNYGJ-QKAIHBBZSA-M | |
Source | PubChem | |
URL | https://pubchem.ncbi.nlm.nih.gov | |
Description | Data deposited in or computed by PubChem | |
Canonical SMILES |
C1C2C(C(C(O2)N3C4=NC=NC(=C4N=C3Br)N)O)OP(=S)(O1)[O-].[Na+] | |
Source | PubChem | |
URL | https://pubchem.ncbi.nlm.nih.gov | |
Description | Data deposited in or computed by PubChem | |
Isomeric SMILES |
C1[C@@H]2[C@H]([C@H]([C@@H](O2)N3C4=NC=NC(=C4N=C3Br)N)O)OP(=S)(O1)[O-].[Na+] | |
Source | PubChem | |
URL | https://pubchem.ncbi.nlm.nih.gov | |
Description | Data deposited in or computed by PubChem | |
Molecular Formula |
C10H10BrN5NaO5PS | |
Source | PubChem | |
URL | https://pubchem.ncbi.nlm.nih.gov | |
Description | Data deposited in or computed by PubChem | |
Molecular Weight |
446.15 g/mol | |
Source | PubChem | |
URL | https://pubchem.ncbi.nlm.nih.gov | |
Description | Data deposited in or computed by PubChem | |
Retrosynthesis Analysis
AI-Powered Synthesis Planning: Our tool employs the Template_relevance Pistachio, Template_relevance Bkms_metabolic, Template_relevance Pistachio_ringbreaker, Template_relevance Reaxys, Template_relevance Reaxys_biocatalysis model, leveraging a vast database of chemical reactions to predict feasible synthetic routes.
One-Step Synthesis Focus: Specifically designed for one-step synthesis, it provides concise and direct routes for your target compounds, streamlining the synthesis process.
Accurate Predictions: Utilizing the extensive PISTACHIO, BKMS_METABOLIC, PISTACHIO_RINGBREAKER, REAXYS, REAXYS_BIOCATALYSIS database, our tool offers high-accuracy predictions, reflecting the latest in chemical research and data.
Strategy Settings
Precursor scoring | Relevance Heuristic |
---|---|
Min. plausibility | 0.01 |
Model | Template_relevance |
Template Set | Pistachio/Bkms_metabolic/Pistachio_ringbreaker/Reaxys/Reaxys_biocatalysis |
Top-N result to add to graph | 6 |
Feasible Synthetic Routes
Q & A
Q1: How does Rp-8-Bromo-Cyclic AMPS affect the formation of syncytiotrophoblasts from cytotrophoblasts?
A1: Rp-8-Bromo-Cyclic AMPS acts as an antagonist of cyclic AMP (cAMP). [] The research demonstrates that the addition of cAMP analogs promotes syncytiotrophoblast formation, evidenced by the loss of E-cadherin and desmoplakin, markers of cell-cell adhesion, and an increase in the number of nuclei per syncytium. [] Conversely, Rp-8-Bromo-Cyclic AMPS and H-89 (a cAMP-dependent protein kinase catalytic subunit inhibitor) hinder cell fusion, suggesting that cAMP signaling pathways are crucial for syncytiotrophoblast formation. []
Disclaimer and Information on In-Vitro Research Products
Please be aware that all articles and product information presented on BenchChem are intended solely for informational purposes. The products available for purchase on BenchChem are specifically designed for in-vitro studies, which are conducted outside of living organisms. In-vitro studies, derived from the Latin term "in glass," involve experiments performed in controlled laboratory settings using cells or tissues. It is important to note that these products are not categorized as medicines or drugs, and they have not received approval from the FDA for the prevention, treatment, or cure of any medical condition, ailment, or disease. We must emphasize that any form of bodily introduction of these products into humans or animals is strictly prohibited by law. It is essential to adhere to these guidelines to ensure compliance with legal and ethical standards in research and experimentation.