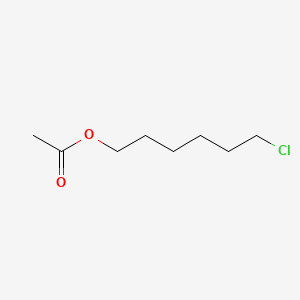
6-Chlorohexyl acetate
Overview
Description
6-Chlorohexyl acetate is an organochlorine ester synthesized via acetylation of 6-chloro-1-hexanol. The standard synthesis involves reacting 6-chloro-1-hexanol with acetic anhydride in the presence of triethylamine (Et₃N) and dimethylaminopyridine (DMAP) as catalysts in dichloromethane (DCM), yielding a pale yellow oil with a high purity (94%) . This compound serves as a key intermediate in organic synthesis, particularly in the preparation of pH-responsive polymers and macrocyclic kinase inhibitors . Its structure features a six-carbon alkyl chain with a terminal chloro group and an acetylated hydroxyl group, conferring both lipophilic and reactive properties.
Preparation Methods
Synthetic Routes for 6-Chlorohexyl Acetate
Esterification of 6-Chloro-1-Hexanol
The most common laboratory and industrial method for preparing this compound is the esterification of 6-chloro-1-hexanol with acetic anhydride or acetic acid derivatives. The reaction is typically catalyzed by pyridine or sulfuric acid and carried out in anhydrous solvents such as dry ethyl ether.
- React 6-chloro-1-hexanol with acetic anhydride in the presence of pyridine.
- Stir the mixture at room temperature for approximately one hour.
- Wash the reaction mixture sequentially with water and saturated brine.
- Dry over anhydrous sodium sulfate.
- Filter and evaporate the solvent.
- Purify the product by distillation to obtain this compound with high purity.
Glycoside-Based Synthesis Involving 6-Chlorohexyl Intermediates
In carbohydrate chemistry, this compound derivatives are synthesized as intermediates for complex glycoside formation. One detailed synthesis involves:
- Starting from a known chlorohexyl glucoside peracetate.
- Deacetylation using sodium methoxide in methanol.
- Formation of benzylidene acetal via reaction with benzaldehyde dimethyl acetal catalyzed by camphorsulfonic acid.
- Chloroacetylation of the resulting alcohol intermediate.
- Reductive opening of the benzylidene acetal using sodium cyanoborohydride and HCl·Et2O in anhydrous tetrahydrofuran at 0 °C, yielding the 6-chlorohexyl acceptor intermediate.
- This intermediate can then be acetylated to form this compound derivatives used for further glycosylation reactions.
Reaction Conditions and Optimization
Step | Reagents/Conditions | Temperature | Time | Yield (%) | Notes |
---|---|---|---|---|---|
Deacetylation | NaOMe/MeOH | Room temperature | 1-2 hours | High | Removes acetyl groups from peracetate |
Benzylidene acetal formation | Benzaldehyde dimethyl acetal, CSA catalyst | Room temperature | Several hours | High | Protects diol groups |
Chloroacetylation | Chloroacetyl chloride or equivalent | 0 °C to RT | 1-3 hours | Moderate to high | Introduces chloroacetyl group |
Reductive opening | NaCNBH3, HCl·Et2O, anhydrous THF | 0 °C | 1-2 hours | High | Opens benzylidene acetal selectively |
Esterification (acetylation) | Acetic anhydride, pyridine or acid catalyst | Room temperature | 1 hour | High | Forms this compound |
This table summarizes key steps in the preparation of this compound derivatives as reported in carbohydrate synthesis literature.
Industrial Production Considerations
Industrial synthesis of this compound generally follows the esterification route but on a larger scale with process optimizations:
- Use of large-scale reactors with controlled temperature and stirring.
- Continuous distillation units to purify the product efficiently.
- Careful control of stoichiometry and reaction time to maximize yield and minimize byproducts.
- Use of dry solvents and inert atmosphere to prevent hydrolysis and side reactions.
- Post-reaction washing and drying steps to ensure removal of residual acids and catalysts.
Nucleophilic Substitution and Derivatization
This compound’s chlorine atom is a reactive site for nucleophilic substitution, enabling the synthesis of various derivatives such as azidohexyl, acetylthiohexyl, and thiobenzylhexyl analogues. For example:
- Nucleophilic displacement of chlorine with sodium azide in dimethylformamide at 80 °C quantitatively yields 6-azidohexyl acetate derivatives.
- Reaction with benzylthiol and sodium hydride in DMF at 80 °C produces 6-benzylthiohexyl acetate derivatives in excellent yield.
These transformations are critical for producing functionalized intermediates for further synthetic applications.
Analytical Characterization
The purity and structure of this compound and its derivatives are confirmed by:
- Nuclear Magnetic Resonance (NMR) Spectroscopy: Characteristic chemical shifts for acetate methyl protons (~2.29 ppm in 1H NMR) and carbonyl carbons (~170 ppm in 13C NMR).
- Gas Chromatography (GC): To assess purity and detect volatile impurities.
- Mass Spectrometry (MS): To confirm molecular weight and fragmentation patterns.
- Infrared Spectroscopy (IR): To verify ester functional groups (carbonyl stretch around 1740 cm⁻¹).
- Elemental Analysis: To confirm chlorine content and overall composition.
Summary Table of Preparation Methods
Research Findings and Notes
- Microwave irradiation can accelerate glycosylation reactions involving 6-chlorohexyl intermediates, reducing reaction time from hours to minutes without compromising yield.
- The choice of protecting groups and reaction conditions in carbohydrate syntheses involving this compound derivatives is critical to avoid side reactions and ensure selective functionalization.
- Industrial scale-up requires optimization of solvent removal and purification steps to maintain product quality and minimize environmental impact.
- The chlorine atom’s reactivity enables diverse functionalizations, expanding the compound’s utility in synthetic organic chemistry.
Chemical Reactions Analysis
Types of Reactions: 6-Chlorohexyl acetate undergoes various chemical reactions, including:
Substitution Reactions: The chlorine atom in this compound can be substituted by other nucleophiles.
Hydrolysis: The ester bond in this compound can be hydrolyzed to produce 6-chlorohexanol and acetic acid.
Common Reagents and Conditions:
Substitution Reactions: Common reagents include nucleophiles such as sodium hydroxide or potassium hydroxide.
Hydrolysis: Acidic or basic conditions can be used for hydrolysis, with common reagents being hydrochloric acid or sodium hydroxide.
Major Products Formed:
Substitution Reactions: Depending on the nucleophile used, products can include various substituted hexyl acetates.
Hydrolysis: The major products are 6-chlorohexanol and acetic acid.
Scientific Research Applications
Chemistry
6-Chlorohexyl acetate serves as a versatile solvent in several chemical reactions. It is particularly useful in:
- Synthesis of Complex Organic Molecules : Acting as an intermediate in the synthesis of more complex compounds.
- Nucleophilic Substitution Reactions : The chlorine atom can be substituted by other nucleophiles, facilitating the formation of various derivatives .
Biology
In biological research, this compound is utilized for:
- Synthesis of Biologically Active Compounds : It plays a role in the development of compounds that exhibit biological activity, which can be crucial for drug discovery.
- Drug Delivery Systems : Ongoing research investigates its potential as a vehicle for delivering therapeutic agents, particularly in controlled release systems .
Medicine
The compound's unique structure allows for exploration in medicinal chemistry:
- Potential Therapeutic Applications : Research is focused on its use in drug formulation and delivery, particularly in systems designed to respond to physiological changes (e.g., pH-responsive systems) that can enhance drug efficacy .
Industrial Applications
This compound finds extensive use in various industrial sectors:
- Production of Coatings and Inks : Its properties make it suitable for formulating paints, coatings, and inks due to its solvent capabilities.
- Cleaning Products : It is also employed in the formulation of cleaners and degreasers, benefiting from its solvent properties.
Case Study 1: Synthesis of Macrocycles
In a study published by the Royal Society of Chemistry, this compound was used as a linker in the synthesis of macrocyclic compounds aimed at targeting epidermal growth factor receptors (EGFR) in cancer therapy. The study demonstrated successful incorporation of this compound into complex organic frameworks, showcasing its utility in medicinal chemistry .
Case Study 2: Controlled Release Systems
Research conducted on polymeric systems utilizing this compound highlighted its role in creating pH-responsive drug delivery vehicles. The study showed that polymers synthesized with this compound could encapsulate DNA and release it under specific conditions, indicating potential applications in gene therapy and targeted drug delivery .
Mechanism of Action
The mechanism of action of 6-chlorohexyl acetate involves its interaction with various molecular targets. The chlorine atom in the compound can participate in nucleophilic substitution reactions, while the ester bond can undergo hydrolysis. These reactions can lead to the formation of different products, which can then interact with biological molecules and pathways.
Comparison with Similar Compounds
The following analysis compares 6-chlorohexyl acetate with structurally or functionally related compounds, emphasizing differences in synthesis, reactivity, and applications.
Hexyl Acetate (CAS 142-92-7)
Structural Similarities : Both compounds share an esterified six-carbon chain.
Key Differences :
- Functional Groups : Hexyl acetate lacks the terminal chloro group, reducing its electrophilic reactivity.
- Applications : Hexyl acetate is primarily used as a solvent in coatings and fragrances, whereas this compound is a specialized intermediate in polymer and pharmaceutical synthesis .
- Synthesis: Hexyl acetate is synthesized via direct esterification of hexanol and acetic acid, avoiding the need for chloro-substituted precursors .
Chloroethyl Acetate
Structural Similarities : Both contain a chloro group and acetate moiety.
Key Differences :
- Chain Length : Chloroethyl acetate has a two-carbon chain, making it more volatile and less thermally stable.
- Reactivity : The shorter chain limits its utility in multi-step syntheses compared to this compound .
Ethyl 6-Chloro-6-Oxohexanoate
Structural Similarities : Both have a six-carbon backbone with a chloro group.
Key Differences :
- Functional Groups : The presence of a keto group at position 6 enhances its reactivity in nucleophilic additions, unlike this compound, which is inert to such reactions.
- Applications: Ethyl 6-chloro-6-oxohexanoate is used in synthesizing enzyme inhibitors, while this compound is tailored for polymer modifications .
Acetamide Derivatives with 6-Chlorohexyl Groups
Example: Acetamide, 2-((6-chlorohexyl)methylamino)-N-(2,6-dimethylphenyl)-, monohydrochloride Structural Similarities: Shares the 6-chlorohexyl moiety. Key Differences:
- Functional Groups : The acetamide and aromatic amine groups enable specific biological interactions (e.g., anti-inflammatory activity), unlike the ester functionality of this compound .
- Synthetic Complexity : Requires additional steps for amidation and aromatic substitution .
Comparative Data Table
Key Research Findings
- Reactivity : The terminal chloro group in this compound allows nucleophilic substitution reactions, enabling its use in synthesizing imidazole derivatives (e.g., 6-(1H-imidazol-1-yl)-hexan-1-ol) for pH-responsive polymers .
- Pharmaceutical Utility: In kinase inhibitor synthesis, this compound acts as a linker, enhancing molecular stability compared to non-chlorinated analogs .
- Thermal Stability : Longer carbon chains in this compound improve thermal stability over shorter analogs like chloroethyl acetate, making it suitable for high-temperature reactions .
Biological Activity
6-Chlorohexyl acetate (CAS #: 40200-18-8) is a synthetic organic compound that has garnered attention for its potential biological activities. This article aims to provide a comprehensive overview of the biological activity of this compound, including its mechanisms of action, case studies, and relevant research findings.
This compound is characterized by the following chemical properties:
Property | Value |
---|---|
Molecular Formula | C₈H₁₅ClO₂ |
Molecular Weight | 174.66 g/mol |
Boiling Point | 215 °C |
Density | 1.04 g/cm³ |
Melting Point | -20 °C |
The biological activity of this compound is primarily attributed to its interactions at the cellular level. Research indicates that halogenated compounds can enhance biological activity through various mechanisms:
- Antimicrobial Activity : Studies have shown that halogenation can improve the antimicrobial properties of compounds by altering their membrane permeability and enhancing their interaction with microbial targets .
- Anticancer Potential : The compound may exhibit anticancer properties by inducing apoptosis in cancer cells and inhibiting cell proliferation. The presence of chlorine in the structure is believed to play a role in modulating these effects .
- Interaction with Enzymes : The compound may act as an inhibitor or modulator of specific enzymes involved in metabolic pathways, which can lead to altered cellular responses and biological outcomes .
Antimicrobial Studies
A study explored the antimicrobial efficacy of various halogenated compounds, including this compound, against different bacterial strains. The results indicated that this compound exhibited significant antibacterial activity, particularly against Gram-positive bacteria. The minimum inhibitory concentration (MIC) was determined to be lower than that of non-halogenated analogs, suggesting enhanced efficacy due to halogenation .
Anticancer Activity
Research focusing on the anticancer properties of halogenated compounds has highlighted that this compound can induce apoptosis in human cancer cell lines. In vitro assays demonstrated a dose-dependent increase in cell death, with IC50 values indicating effective concentrations for therapeutic applications . The mechanism involved the activation of caspase pathways and inhibition of cell cycle progression.
Toxicological Assessment
Toxicological studies are crucial for evaluating the safety profile of this compound. In animal models, acute toxicity tests revealed that the compound has a moderate toxicity profile, with no significant adverse effects observed at lower doses. However, higher concentrations led to liver and kidney stress, necessitating further investigation into its safety for human use .
Q & A
Basic Research Questions
Q. What are the optimal conditions for synthesizing 6-Chlorohexyl acetate with high yield and purity?
- Methodology : The synthesis involves reacting 6-chloro-1-hexanol with acetic anhydride in dichloromethane (DCM) using triethylamine (Et₃N) as a base and dimethylaminopyridine (DMAP) as a catalyst. The reaction proceeds at room temperature, followed by purification via standard aqueous workup (e.g., washing with NaHCO₃ and brine) to yield 94% of the product as a pale yellow oil. Key factors include stoichiometric excess of acetic anhydride (1.5:1 molar ratio to alcohol) and catalytic DMAP to accelerate acetylation .
Q. What analytical techniques are recommended for confirming the structural integrity of this compound?
- Methodology : Nuclear Magnetic Resonance (NMR) spectroscopy (¹H and ¹³C) is critical for verifying the acetate and chlorohexyl moieties. Mass spectrometry (MS) confirms molecular weight (e.g., via ESI or EI-MS). Purity can be assessed using gas chromatography (GC) or HPLC with UV detection. For derivatives like phosphonates, ³¹P NMR provides additional structural insights .
Q. How should this compound be stored to ensure stability during experimental workflows?
- Methodology : Store in a cool, dry environment (<4°C) under inert gas (e.g., argon) to prevent hydrolysis of the chlorohexyl group. Use amber glass vials to minimize light-induced degradation. Stability tests via periodic GC or NMR analysis are recommended for long-term storage .
Advanced Research Questions
Q. What are the mechanistic implications of using this compound in nucleophilic substitution reactions (e.g., with imidazole)?
- Methodology : The chlorohexyl group undergoes SN2 reactions with nucleophiles (e.g., imidazole) under basic conditions (e.g., NaH in DMSO). Kinetic studies show higher reactivity compared to shorter-chain analogs (e.g., 4-chlorobutyl acetate) due to reduced steric hindrance. Reaction progress can be monitored via TLC or in situ FTIR to track carbonyl or imidazole incorporation .
Q. How does the chlorohexyl substituent influence reaction outcomes in glycosylation processes compared to azidohexyl groups?
- Methodology : In glycosylation reactions, 6-chlorohexyl aglycons improve coupling efficiency (e.g., galactosyl imidate at 40°C under BF₃·OEt₂ activation) compared to azidohexyl analogs. The chloro group’s electron-withdrawing nature stabilizes transition states, while azido groups may introduce steric or electronic mismatches. Post-reaction conversion to aminohexyl derivatives via azide reduction is also streamlined .
Q. What challenges arise in characterizing α-amino phosphonate derivatives of this compound, and how can they be resolved?
- Methodology : Derivatives like Diethyl (((6-chlorohexyl)amino)carbonyl)phosphonate require multi-nuclear NMR (¹H, ¹³C, ³¹P) for full characterization. Challenges include signal overlap in crowded spectral regions (e.g., δ 1.0–2.5 ppm for hexyl chains). High-resolution MS and X-ray crystallography (if crystals form) resolve ambiguities. Reaction optimization (e.g., solvent polarity, temperature) minimizes by-products like desulfurization artifacts .
Q. What are the common contradictions in reported reaction mechanisms involving this compound derivatives?
- Case Study : While reports superior glycosylation efficiency with chlorohexyl groups, other studies note competing elimination pathways in strongly basic conditions. Contradictions may arise from solvent choice (DMSO vs. THF) or nucleophile strength. Systematic kinetic profiling and DFT calculations can reconcile discrepancies .
Q. How can this compound be integrated into pH-responsive block copolymers for targeted drug delivery?
- Methodology : The chlorohexyl group serves as a hydrophobic segment in copolymer design (e.g., with imidazole-containing blocks). Self-assembly into micelles in aqueous media (pH 5–7) enables nucleic acid encapsulation. Stability and release kinetics are tunable via copolymer composition and crosslinking density. In vitro cell entry efficiency is validated using fluorescence-tagged constructs .
Q. Data Contradictions and Resolution Strategies
Properties
IUPAC Name |
6-chlorohexyl acetate | |
---|---|---|
Source | PubChem | |
URL | https://pubchem.ncbi.nlm.nih.gov | |
Description | Data deposited in or computed by PubChem | |
InChI |
InChI=1S/C8H15ClO2/c1-8(10)11-7-5-3-2-4-6-9/h2-7H2,1H3 | |
Source | PubChem | |
URL | https://pubchem.ncbi.nlm.nih.gov | |
Description | Data deposited in or computed by PubChem | |
InChI Key |
AHEBJCQIQYFSGF-UHFFFAOYSA-N | |
Source | PubChem | |
URL | https://pubchem.ncbi.nlm.nih.gov | |
Description | Data deposited in or computed by PubChem | |
Canonical SMILES |
CC(=O)OCCCCCCCl | |
Source | PubChem | |
URL | https://pubchem.ncbi.nlm.nih.gov | |
Description | Data deposited in or computed by PubChem | |
Molecular Formula |
C8H15ClO2 | |
Source | PubChem | |
URL | https://pubchem.ncbi.nlm.nih.gov | |
Description | Data deposited in or computed by PubChem | |
DSSTOX Substance ID |
DTXSID00885798 | |
Record name | 1-Hexanol, 6-chloro-, 1-acetate | |
Source | EPA DSSTox | |
URL | https://comptox.epa.gov/dashboard/DTXSID00885798 | |
Description | DSSTox provides a high quality public chemistry resource for supporting improved predictive toxicology. | |
Molecular Weight |
178.65 g/mol | |
Source | PubChem | |
URL | https://pubchem.ncbi.nlm.nih.gov | |
Description | Data deposited in or computed by PubChem | |
CAS No. |
40200-18-8 | |
Record name | 1-Hexanol, 6-chloro-, 1-acetate | |
Source | CAS Common Chemistry | |
URL | https://commonchemistry.cas.org/detail?cas_rn=40200-18-8 | |
Description | CAS Common Chemistry is an open community resource for accessing chemical information. Nearly 500,000 chemical substances from CAS REGISTRY cover areas of community interest, including common and frequently regulated chemicals, and those relevant to high school and undergraduate chemistry classes. This chemical information, curated by our expert scientists, is provided in alignment with our mission as a division of the American Chemical Society. | |
Explanation | The data from CAS Common Chemistry is provided under a CC-BY-NC 4.0 license, unless otherwise stated. | |
Record name | 1-Hexanol, 6-chloro-, 1-acetate | |
Source | ChemIDplus | |
URL | https://pubchem.ncbi.nlm.nih.gov/substance/?source=chemidplus&sourceid=0040200188 | |
Description | ChemIDplus is a free, web search system that provides access to the structure and nomenclature authority files used for the identification of chemical substances cited in National Library of Medicine (NLM) databases, including the TOXNET system. | |
Record name | 1-Hexanol, 6-chloro-, 1-acetate | |
Source | EPA Chemicals under the TSCA | |
URL | https://www.epa.gov/chemicals-under-tsca | |
Description | EPA Chemicals under the Toxic Substances Control Act (TSCA) collection contains information on chemicals and their regulations under TSCA, including non-confidential content from the TSCA Chemical Substance Inventory and Chemical Data Reporting. | |
Record name | 1-Hexanol, 6-chloro-, 1-acetate | |
Source | EPA DSSTox | |
URL | https://comptox.epa.gov/dashboard/DTXSID00885798 | |
Description | DSSTox provides a high quality public chemistry resource for supporting improved predictive toxicology. | |
Record name | 6-chlorohexyl acetate | |
Source | European Chemicals Agency (ECHA) | |
URL | https://echa.europa.eu/substance-information/-/substanceinfo/100.049.834 | |
Description | The European Chemicals Agency (ECHA) is an agency of the European Union which is the driving force among regulatory authorities in implementing the EU's groundbreaking chemicals legislation for the benefit of human health and the environment as well as for innovation and competitiveness. | |
Explanation | Use of the information, documents and data from the ECHA website is subject to the terms and conditions of this Legal Notice, and subject to other binding limitations provided for under applicable law, the information, documents and data made available on the ECHA website may be reproduced, distributed and/or used, totally or in part, for non-commercial purposes provided that ECHA is acknowledged as the source: "Source: European Chemicals Agency, http://echa.europa.eu/". Such acknowledgement must be included in each copy of the material. ECHA permits and encourages organisations and individuals to create links to the ECHA website under the following cumulative conditions: Links can only be made to webpages that provide a link to the Legal Notice page. | |
Synthesis routes and methods I
Procedure details
Synthesis routes and methods II
Procedure details
Retrosynthesis Analysis
AI-Powered Synthesis Planning: Our tool employs the Template_relevance Pistachio, Template_relevance Bkms_metabolic, Template_relevance Pistachio_ringbreaker, Template_relevance Reaxys, Template_relevance Reaxys_biocatalysis model, leveraging a vast database of chemical reactions to predict feasible synthetic routes.
One-Step Synthesis Focus: Specifically designed for one-step synthesis, it provides concise and direct routes for your target compounds, streamlining the synthesis process.
Accurate Predictions: Utilizing the extensive PISTACHIO, BKMS_METABOLIC, PISTACHIO_RINGBREAKER, REAXYS, REAXYS_BIOCATALYSIS database, our tool offers high-accuracy predictions, reflecting the latest in chemical research and data.
Strategy Settings
Precursor scoring | Relevance Heuristic |
---|---|
Min. plausibility | 0.01 |
Model | Template_relevance |
Template Set | Pistachio/Bkms_metabolic/Pistachio_ringbreaker/Reaxys/Reaxys_biocatalysis |
Top-N result to add to graph | 6 |
Feasible Synthetic Routes
Disclaimer and Information on In-Vitro Research Products
Please be aware that all articles and product information presented on BenchChem are intended solely for informational purposes. The products available for purchase on BenchChem are specifically designed for in-vitro studies, which are conducted outside of living organisms. In-vitro studies, derived from the Latin term "in glass," involve experiments performed in controlled laboratory settings using cells or tissues. It is important to note that these products are not categorized as medicines or drugs, and they have not received approval from the FDA for the prevention, treatment, or cure of any medical condition, ailment, or disease. We must emphasize that any form of bodily introduction of these products into humans or animals is strictly prohibited by law. It is essential to adhere to these guidelines to ensure compliance with legal and ethical standards in research and experimentation.