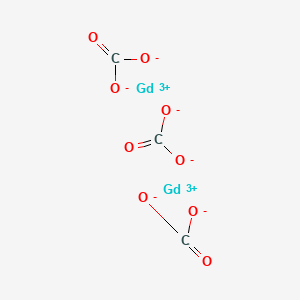
Gadolinium carbonate
Overview
Description
Gadolinium carbonate is a chemical compound with the formula Gd₂(CO₃)₃. It is a white, water-insoluble powder that is primarily used in various scientific and industrial applications. Gadolinium, the central element in this compound, is a rare-earth metal known for its unique magnetic and optical properties .
Mechanism of Action
Target of Action
Gadolinium carbonate primarily targets protons in the human body . The gadolinium ion is useful as an MRI contrast agent because it has seven unpaired electrons, which is the greatest number of unpaired electron spins possible for an atom . These protons are abundant in the body due to high fat and water content .
Mode of Action
The mode of action of this compound is based on the behavior of protons when placed in a strong magnetic field, which is interpreted and transformed into images by magnetic resonance (MR) instruments . MR images are based primarily on proton density and proton relaxation dynamics . Gadolinium molecules shorten the spin-lattice relaxation time (T1) of voxels in which they are present .
Biochemical Pathways
Several signaling pathways have been implicated in Gd (III) mechanisms of toxicity, such as MAPK/ERK (mitogen-activated protein kinase/extracellular signal-regulated kinase), PI3K/Akt (phosphoinositide-3-kinase/protein kinase B), and EGFR (epidermal growth factor receptor) signaling . These pathways suggest that Gd (III) interferes with the transduction of molecules involved in the regulation of inflammatory processes, and in cell metabolism, proliferation, growth, and survival .
Pharmacokinetics
Gadolinium-based contrast agents (GBCAs), such as this compound, are predominantly removed renally without metabolization . Approximately 90% of administered GBCA is excreted in the urine within 24 hours in patients with normal renal function . An understanding of the pharmacokinetics in humans and animals alike are pivotal to the understanding of the distribution and excretion of gadolinium and GBCAs, and ultimately their potential retention .
Result of Action
The primary result of this compound’s action is the enhancement of magnetic resonance imaging (MRI), a crucial tool for medical diagnosis and treatment monitoring across multiple clinical settings . Studies have shown that exposure to gbcas is associated with gadolinium release and tissue deposition that may cause short- and long-term toxicity in several organs, including the kidney, the main excretion organ of most gbcas .
Action Environment
The action, efficacy, and stability of this compound can be influenced by environmental factors. For instance, the structural integrity of chelates are susceptible to ultraviolet light, regardless of configuration . Furthermore, the accumulation of gadolinium in our environment is being addressed with increasing urgency since the second decade of the 21st century; recent studies show anthropogenic gadolinium to be lurking in our wastewaters, crops, tap water, and even marine wildlife .
Biochemical Analysis
Biochemical Properties
Gadolinium carbonate plays a significant role in biochemical reactions, particularly in the context of its use as a contrast agent in magnetic resonance imaging (MRI). The compound interacts with various enzymes, proteins, and other biomolecules. For instance, gadolinium ions (Gd3+) can bind to proteins and enzymes, altering their structure and function. This interaction is crucial for enhancing the contrast in MRI scans, as the paramagnetic properties of gadolinium ions increase the relaxation rates of water protons in the vicinity, thereby improving image clarity .
Cellular Effects
This compound has notable effects on various types of cells and cellular processes. It influences cell function by affecting cell signaling pathways, gene expression, and cellular metabolism. For example, gadolinium ions can interfere with calcium channels, leading to altered calcium signaling within cells. This disruption can impact processes such as muscle contraction, neurotransmitter release, and cell proliferation . Additionally, this compound has been shown to induce oxidative stress and apoptosis in certain cell types, further highlighting its impact on cellular function .
Molecular Mechanism
The molecular mechanism of this compound involves its interaction with biomolecules at the molecular level. Gadolinium ions can bind to various proteins and enzymes, leading to inhibition or activation of their functions. For instance, gadolinium ions can inhibit calcium-dependent enzymes by competing with calcium ions for binding sites. This inhibition can result in altered cellular processes and signaling pathways . Furthermore, this compound can induce changes in gene expression by affecting transcription factors and other regulatory proteins .
Temporal Effects in Laboratory Settings
In laboratory settings, the effects of this compound can change over time. The stability and degradation of the compound are critical factors that influence its long-term effects on cellular function. Studies have shown that this compound can degrade into gadolinium ions and carbonate ions over time, which can impact its efficacy and safety as a contrast agent . Long-term exposure to this compound has been associated with nephrogenic systemic fibrosis in patients with impaired renal function, highlighting the importance of monitoring its temporal effects .
Dosage Effects in Animal Models
The effects of this compound vary with different dosages in animal models. At low doses, this compound can enhance MRI contrast without causing significant toxicity. At higher doses, the compound can induce toxic effects, such as nephrotoxicity and hepatotoxicity . Studies have also shown that this compound can accumulate in various organs, including the brain, liver, and kidneys, leading to potential adverse effects . It is crucial to determine the optimal dosage to balance efficacy and safety.
Metabolic Pathways
This compound is involved in several metabolic pathways, primarily related to its excretion and clearance from the body. The compound is primarily excreted through the kidneys, with a high fraction excreted in urine without metabolic modification . The involvement of this compound in metabolic pathways can influence metabolic flux and metabolite levels, particularly in patients with renal impairment . Understanding these pathways is essential for assessing the safety and efficacy of this compound as a contrast agent.
Transport and Distribution
The transport and distribution of this compound within cells and tissues are critical for its function as a contrast agent. Gadolinium ions can be transported across cell membranes through various transporters and binding proteins. Once inside the cells, gadolinium ions can localize to specific cellular compartments, such as the cytoplasm and nucleus . The distribution of this compound within tissues can also affect its accumulation and localization, influencing its efficacy and potential toxicity .
Subcellular Localization
The subcellular localization of this compound is essential for understanding its activity and function. Gadolinium ions can localize to specific organelles, such as lysosomes and mitochondria, where they can exert their effects . The targeting signals and post-translational modifications of proteins can direct gadolinium ions to these compartments, influencing their activity and function. For instance, gadolinium ions can induce oxidative stress and apoptosis by disrupting mitochondrial function .
Preparation Methods
Synthetic Routes and Reaction Conditions: Gadolinium carbonate can be synthesized through the reaction of gadolinium chloride (GdCl₃) with sodium carbonate (Na₂CO₃) in an aqueous solution. The reaction typically occurs at room temperature and results in the precipitation of this compound: [ 2 \text{GdCl}_3 + 3 \text{Na}_2\text{CO}_3 \rightarrow \text{Gd}_2(\text{CO}_3)_3 + 6 \text{NaCl} ]
Industrial Production Methods: Industrial production of this compound often involves the same basic reaction but on a larger scale. The process may include additional steps such as filtration, washing, and drying to obtain a high-purity product .
Chemical Reactions Analysis
Types of Reactions: Gadolinium carbonate undergoes several types of chemical reactions, including:
-
Decomposition: When heated, this compound decomposes to form gadolinium oxide (Gd₂O₃) and carbon dioxide (CO₂). [ \text{Gd}_2(\text{CO}_3)_3 \rightarrow \text{Gd}_2\text{O}_3 + 3 \text{CO}_2 ]
-
Reaction with Acids: this compound reacts with acids to form gadolinium salts and carbon dioxide. [ \text{Gd}_2(\text{CO}_3)_3 + 6 \text{HCl} \rightarrow 2 \text{GdCl}_3 + 3 \text{CO}_2 + 3 \text{H}_2\text{O} ]
Common Reagents and Conditions:
Acids: Hydrochloric acid (HCl), sulfuric acid (H₂SO₄)
Conditions: Room temperature, aqueous solutions
Major Products Formed:
- Gadolinium oxide (Gd₂O₃)
- Gadolinium chloride (GdCl₃)
- Carbon dioxide (CO₂)
Scientific Research Applications
Gadolinium carbonate has a wide range of applications in scientific research, including:
- Chemistry: Used as a precursor for the synthesis of other gadolinium compounds.
- Biology: Employed in the preparation of gadolinium-based contrast agents for magnetic resonance imaging (MRI).
- Medicine: Utilized in the development of MRI contrast agents due to its paramagnetic properties.
- Industry: Applied in the manufacturing of phosphors for color television tubes and in the production of specialized glass and ceramics .
Comparison with Similar Compounds
Gadolinium carbonate can be compared with other gadolinium compounds such as gadolinium oxide (Gd₂O₃) and gadolinium chloride (GdCl₃). While all these compounds contain gadolinium, they differ in their chemical properties and applications:
- Gadolinium Oxide (Gd₂O₃): Primarily used in the production of phosphors and as a catalyst in various chemical reactions.
- Gadolinium Chloride (GdCl₃): Commonly used as a precursor for the synthesis of other gadolinium compounds and in the preparation of gadolinium-based contrast agents .
Each of these compounds has unique properties that make them suitable for specific applications, highlighting the versatility of gadolinium in scientific and industrial fields.
Properties
CAS No. |
5895-49-8 |
---|---|
Molecular Formula |
CH2GdO3 |
Molecular Weight |
219.3 g/mol |
IUPAC Name |
carbonic acid;gadolinium |
InChI |
InChI=1S/CH2O3.Gd/c2-1(3)4;/h(H2,2,3,4); |
InChI Key |
RCSUUJCNLXUZTA-UHFFFAOYSA-N |
SMILES |
C(=O)([O-])[O-].C(=O)([O-])[O-].C(=O)([O-])[O-].[Gd+3].[Gd+3] |
Canonical SMILES |
C(=O)(O)O.[Gd] |
Key on ui other cas no. |
5895-49-8 |
Origin of Product |
United States |
Retrosynthesis Analysis
AI-Powered Synthesis Planning: Our tool employs the Template_relevance Pistachio, Template_relevance Bkms_metabolic, Template_relevance Pistachio_ringbreaker, Template_relevance Reaxys, Template_relevance Reaxys_biocatalysis model, leveraging a vast database of chemical reactions to predict feasible synthetic routes.
One-Step Synthesis Focus: Specifically designed for one-step synthesis, it provides concise and direct routes for your target compounds, streamlining the synthesis process.
Accurate Predictions: Utilizing the extensive PISTACHIO, BKMS_METABOLIC, PISTACHIO_RINGBREAKER, REAXYS, REAXYS_BIOCATALYSIS database, our tool offers high-accuracy predictions, reflecting the latest in chemical research and data.
Strategy Settings
Precursor scoring | Relevance Heuristic |
---|---|
Min. plausibility | 0.01 |
Model | Template_relevance |
Template Set | Pistachio/Bkms_metabolic/Pistachio_ringbreaker/Reaxys/Reaxys_biocatalysis |
Top-N result to add to graph | 6 |
Feasible Synthetic Routes
ANone: Gadolinium carbonate, with the molecular formula Gd2(CO3)3, typically exists as a hydrate, often incorporating water molecules within its structure. [, , ] While its exact molecular weight varies based on the degree of hydration, anhydrous this compound possesses a molecular weight of 464.52 g/mol. [, ]
ANone: Several spectroscopic techniques provide valuable insights into the structural and compositional characteristics of this compound. X-ray diffraction (XRD) unveils its crystalline structure and phase purity. [, , , ] Fourier transform infrared spectroscopy (FTIR) helps identify characteristic functional groups, such as carbonyl and hydroxyl groups, and their interactions. [, ] UV-Vis diffuse reflectance spectroscopy (DRS) can be employed to determine the band gap energy of this compound, especially valuable when assessing its photocatalytic potential. []
ANone: The chosen synthesis method significantly influences the physicochemical properties of this compound. For instance, reaction crystallization enables the formation of nanocrystals, with parameters like reactant concentrations, pH, and acoustic energy impacting their size. [] Solvothermal synthesis, often employing polyethylene glycol (PEG), leads to the production of PEGylated this compound particles ((Gd2(CO3)3)@PEG) with varying crystallinity and morphology depending on reaction time. [, ] Microemulsion-based synthesis, utilizing CO2 as a reactant, yields hollow nanospheres with tunable sizes, offering potential for applications like drug delivery. []
ANone: Polyethylene glycol (PEG) plays a crucial role in modifying the surface properties of this compound during solvothermal synthesis. It acts as a capping agent, influencing the size and morphology of the resulting particles. [, ] The presence of PEG on the surface is confirmed by FTIR analysis, which detects characteristic carbonyl and hydroxyl groups. [] This PEGylation can enhance the biocompatibility and stability of this compound, making it suitable for biomedical applications. []
ANone: The unique properties of this compound, particularly in its nanostructured forms, make it a promising candidate for drug delivery and imaging applications. For instance, hollow this compound nanospheres, synthesized via a microemulsion method, can encapsulate anti-cancer drugs like doxorubicin. [] These nanocontainers offer the possibility of multimodal imaging, including optical imaging (OI) and magnetic resonance imaging (MRI), owing to the intrinsic fluorescence of doxorubicin and the magnetic properties of gadolinium. [] Furthermore, the magnetothermal heating capability of these nanospheres presents an intriguing avenue for triggered drug release. []
ANone: Yes, research has shown that both this compound and its thermally decomposed product, gadolinium oxide (Gd2O3), possess photocatalytic activity. [] Studies demonstrate their effectiveness in degrading organic pollutants like methyl orange under UV or visible light irradiation. [] This photocatalytic behavior stems from the electronic structure of these materials and their ability to generate electron-hole pairs upon light absorption, ultimately leading to the degradation of organic pollutants.
ANone: Thermal analysis, often coupled with mass spectrometry (TGA-MS), reveals the thermal behavior of this compound. [] The compound typically starts decomposing at elevated temperatures, releasing carbon dioxide and water, ultimately forming gadolinium oxide (Gd2O3). [, ] The exact decomposition temperature depends on factors such as the degree of hydration and the presence of any surface modifications. This thermal decomposition pathway offers a route for preparing gadolinium oxide nanoparticles with controlled morphology and size. [, ]
ANone: Research highlights the ability of this compound to interact with other materials, leading to the formation of composites or modified structures. It can be combined with silica to create core-shell nanoparticles, where silica acts as a protective layer and allows for surface functionalization. [, ] For instance, fluorescein isothiocyanate (FITC) can be embedded within the silica shell, imparting fluorescent properties to the nanoparticles. [] Such composites demonstrate potential as bi-modal contrast agents for both fluorescence imaging and MRI. [] Additionally, this compound can be integrated with other metal oxides, such as chromium oxide, to synthesize nanocomposites with intriguing dielectric properties. [] These interactions highlight the versatility of this compound in material design and its potential for diverse applications.
Disclaimer and Information on In-Vitro Research Products
Please be aware that all articles and product information presented on BenchChem are intended solely for informational purposes. The products available for purchase on BenchChem are specifically designed for in-vitro studies, which are conducted outside of living organisms. In-vitro studies, derived from the Latin term "in glass," involve experiments performed in controlled laboratory settings using cells or tissues. It is important to note that these products are not categorized as medicines or drugs, and they have not received approval from the FDA for the prevention, treatment, or cure of any medical condition, ailment, or disease. We must emphasize that any form of bodily introduction of these products into humans or animals is strictly prohibited by law. It is essential to adhere to these guidelines to ensure compliance with legal and ethical standards in research and experimentation.