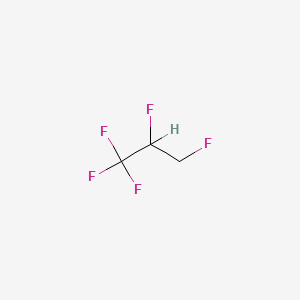
1,1,1,2,3-Pentafluoropropane
Overview
Description
1,1,1,2,3-Pentafluoropropane is a colorless, odorless gas with the molecular formula C3H3F5. It belongs to the family of hydrofluorocarbons (HFCs) and is commonly used in various industrial applications due to its unique properties.
Mechanism of Action
Target of Action
1,1,1,2,3-Pentafluoropropane, also known as HFC-245fa, is primarily used for closed-cell spray foam insulation . It is a hydrofluorocarbon and its primary target is the insulation material where it is used as a blowing agent .
Mode of Action
It is known that it is used as a blowing agent in the production of closed-cell spray foam insulation .
Biochemical Pathways
It is primarily used in industrial applications rather than biological systems .
Result of Action
The result of the action of this compound is the production of closed-cell spray foam insulation . The gas expands when it is applied, creating a foam that provides effective insulation .
Action Environment
The action of this compound is influenced by environmental factors such as temperature and pressure . It is intended to remain trapped within the foam insulation, and it is practically non-biodegradable with a lifetime of 7.2 years when it eventually does escape into the atmosphere . It has a high global warming potential of 950 (950 times the global warming effect of CO2) .
Preparation Methods
Synthetic Routes and Reaction Conditions: 1,1,1,2,3-Pentafluoropropane can be synthesized through the fluorination of propane or its derivatives. The process typically involves the reaction of propane with hydrogen fluoride (HF) in the presence of a catalyst such as antimony pentafluoride (SbF5) under controlled conditions.
Industrial Production Methods: In an industrial setting, the production of this compound is carried out in large-scale reactors where propane and hydrogen fluoride are continuously fed into the system. The reaction is exothermic, and the heat generated is managed through cooling systems to maintain optimal reaction conditions.
Chemical Reactions Analysis
Types of Reactions: 1,1,1,2,3-Pentafluoropropane undergoes various types of chemical reactions, including oxidation, reduction, and substitution reactions.
Common Reagents and Conditions:
Oxidation: Oxidation reactions can be performed using oxidizing agents such as potassium permanganate (KMnO4) or hydrogen peroxide (H2O2) under acidic conditions.
Reduction: Reduction reactions typically involve the use of reducing agents like lithium aluminum hydride (LiAlH4) or sodium borohydride (NaBH4) in anhydrous solvents.
Substitution: Substitution reactions can be carried out using nucleophiles such as halides or amines in the presence of a suitable solvent.
Major Products Formed:
Oxidation: The major products of oxidation reactions include carboxylic acids and ketones.
Reduction: Reduction reactions yield alcohols and amines as major products.
Substitution: Substitution reactions result in the formation of halogenated or aminated derivatives of this compound.
Scientific Research Applications
1,1,1,2,3-Pentafluoropropane is widely used in scientific research due to its unique properties and versatility. Some of its applications include:
Chemistry: It is used as a solvent and reagent in organic synthesis and as a reference standard in spectroscopy.
Biology: It serves as a tracer in biological studies to understand metabolic pathways and enzyme activities.
Medicine: It is utilized in the development of pharmaceuticals and as a component in medical imaging agents.
Industry: It is employed as a refrigerant, propellant, and blowing agent in the production of foamed plastics and other materials.
Comparison with Similar Compounds
1,1,1,2,3-Pentafluoropropane is similar to other hydrofluorocarbons such as 1,1,1,2,2,3,3-heptafluoropropane (HFC-227ea) and 1,1,1,3,3,3-hexafluoropropane (HFC-236fa). it is unique in its specific combination of fluorine atoms, which gives it distinct physical and chemical properties. These properties make it more suitable for certain applications compared to its counterparts.
Does this cover everything you were looking for, or is there something specific you'd like to know more about?
Properties
IUPAC Name |
1,1,1,2,3-pentafluoropropane | |
---|---|---|
Source | PubChem | |
URL | https://pubchem.ncbi.nlm.nih.gov | |
Description | Data deposited in or computed by PubChem | |
InChI |
InChI=1S/C3H3F5/c4-1-2(5)3(6,7)8/h2H,1H2 | |
Source | PubChem | |
URL | https://pubchem.ncbi.nlm.nih.gov | |
Description | Data deposited in or computed by PubChem | |
InChI Key |
ZDCWZRQSHBQRGN-UHFFFAOYSA-N | |
Source | PubChem | |
URL | https://pubchem.ncbi.nlm.nih.gov | |
Description | Data deposited in or computed by PubChem | |
Canonical SMILES |
C(C(C(F)(F)F)F)F | |
Source | PubChem | |
URL | https://pubchem.ncbi.nlm.nih.gov | |
Description | Data deposited in or computed by PubChem | |
Molecular Formula |
C3H3F5 | |
Source | PubChem | |
URL | https://pubchem.ncbi.nlm.nih.gov | |
Description | Data deposited in or computed by PubChem | |
DSSTOX Substance ID |
DTXSID70883382 | |
Record name | 1,1,1,2,3-Pentafluoropropane | |
Source | EPA DSSTox | |
URL | https://comptox.epa.gov/dashboard/DTXSID70883382 | |
Description | DSSTox provides a high quality public chemistry resource for supporting improved predictive toxicology. | |
Molecular Weight |
134.05 g/mol | |
Source | PubChem | |
URL | https://pubchem.ncbi.nlm.nih.gov | |
Description | Data deposited in or computed by PubChem | |
CAS No. |
431-31-2 | |
Record name | 1,1,1,2,3-Pentafluoropropane | |
Source | CAS Common Chemistry | |
URL | https://commonchemistry.cas.org/detail?cas_rn=431-31-2 | |
Description | CAS Common Chemistry is an open community resource for accessing chemical information. Nearly 500,000 chemical substances from CAS REGISTRY cover areas of community interest, including common and frequently regulated chemicals, and those relevant to high school and undergraduate chemistry classes. This chemical information, curated by our expert scientists, is provided in alignment with our mission as a division of the American Chemical Society. | |
Explanation | The data from CAS Common Chemistry is provided under a CC-BY-NC 4.0 license, unless otherwise stated. | |
Record name | Propane, 1,1,1,2,3-pentafluoro- | |
Source | ChemIDplus | |
URL | https://pubchem.ncbi.nlm.nih.gov/substance/?source=chemidplus&sourceid=0000431312 | |
Description | ChemIDplus is a free, web search system that provides access to the structure and nomenclature authority files used for the identification of chemical substances cited in National Library of Medicine (NLM) databases, including the TOXNET system. | |
Record name | Propane, 1,1,1,2,3-pentafluoro- | |
Source | EPA Chemicals under the TSCA | |
URL | https://www.epa.gov/chemicals-under-tsca | |
Description | EPA Chemicals under the Toxic Substances Control Act (TSCA) collection contains information on chemicals and their regulations under TSCA, including non-confidential content from the TSCA Chemical Substance Inventory and Chemical Data Reporting. | |
Record name | 1,1,1,2,3-Pentafluoropropane | |
Source | EPA DSSTox | |
URL | https://comptox.epa.gov/dashboard/DTXSID70883382 | |
Description | DSSTox provides a high quality public chemistry resource for supporting improved predictive toxicology. | |
Record name | 1,1,1,2,3-pentafluoropropane | |
Source | European Chemicals Agency (ECHA) | |
URL | https://echa.europa.eu/information-on-chemicals | |
Description | The European Chemicals Agency (ECHA) is an agency of the European Union which is the driving force among regulatory authorities in implementing the EU's groundbreaking chemicals legislation for the benefit of human health and the environment as well as for innovation and competitiveness. | |
Explanation | Use of the information, documents and data from the ECHA website is subject to the terms and conditions of this Legal Notice, and subject to other binding limitations provided for under applicable law, the information, documents and data made available on the ECHA website may be reproduced, distributed and/or used, totally or in part, for non-commercial purposes provided that ECHA is acknowledged as the source: "Source: European Chemicals Agency, http://echa.europa.eu/". Such acknowledgement must be included in each copy of the material. ECHA permits and encourages organisations and individuals to create links to the ECHA website under the following cumulative conditions: Links can only be made to webpages that provide a link to the Legal Notice page. | |
Synthesis routes and methods I
Procedure details
Synthesis routes and methods II
Procedure details
Synthesis routes and methods III
Procedure details
Retrosynthesis Analysis
AI-Powered Synthesis Planning: Our tool employs the Template_relevance Pistachio, Template_relevance Bkms_metabolic, Template_relevance Pistachio_ringbreaker, Template_relevance Reaxys, Template_relevance Reaxys_biocatalysis model, leveraging a vast database of chemical reactions to predict feasible synthetic routes.
One-Step Synthesis Focus: Specifically designed for one-step synthesis, it provides concise and direct routes for your target compounds, streamlining the synthesis process.
Accurate Predictions: Utilizing the extensive PISTACHIO, BKMS_METABOLIC, PISTACHIO_RINGBREAKER, REAXYS, REAXYS_BIOCATALYSIS database, our tool offers high-accuracy predictions, reflecting the latest in chemical research and data.
Strategy Settings
Precursor scoring | Relevance Heuristic |
---|---|
Min. plausibility | 0.01 |
Model | Template_relevance |
Template Set | Pistachio/Bkms_metabolic/Pistachio_ringbreaker/Reaxys/Reaxys_biocatalysis |
Top-N result to add to graph | 6 |
Feasible Synthetic Routes
Disclaimer and Information on In-Vitro Research Products
Please be aware that all articles and product information presented on BenchChem are intended solely for informational purposes. The products available for purchase on BenchChem are specifically designed for in-vitro studies, which are conducted outside of living organisms. In-vitro studies, derived from the Latin term "in glass," involve experiments performed in controlled laboratory settings using cells or tissues. It is important to note that these products are not categorized as medicines or drugs, and they have not received approval from the FDA for the prevention, treatment, or cure of any medical condition, ailment, or disease. We must emphasize that any form of bodily introduction of these products into humans or animals is strictly prohibited by law. It is essential to adhere to these guidelines to ensure compliance with legal and ethical standards in research and experimentation.