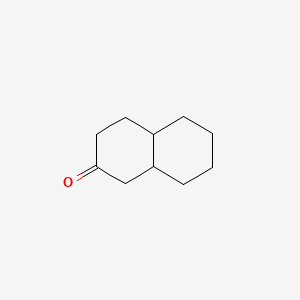
2-DECALONE
Overview
Description
2-DECALONE is an organic compound with the molecular formula C10H16O It is a saturated derivative of naphthalenone, characterized by the presence of a fully hydrogenated naphthalene ring system
Preparation Methods
Synthetic Routes and Reaction Conditions: The synthesis of 2-DECALONE typically involves the hydrogenation of naphthalenone. This process can be carried out using a variety of catalysts, such as palladium on carbon (Pd/C) or platinum oxide (PtO2), under high-pressure hydrogen gas. The reaction conditions often include temperatures ranging from 50°C to 150°C and pressures between 10 to 50 atmospheres .
Industrial Production Methods: In an industrial setting, the production of this compound can be scaled up using continuous flow reactors. These reactors allow for better control over reaction conditions and improved efficiency. The use of robust catalysts and optimized reaction parameters ensures high yields and purity of the final product .
Chemical Reactions Analysis
Types of Reactions: 2-DECALONE undergoes various chemical reactions, including:
Oxidation: This compound can be oxidized to form naphthalenone derivatives using oxidizing agents such as potassium permanganate (KMnO4) or chromium trioxide (CrO3).
Reduction: Further reduction of this compound can lead to the formation of fully saturated hydrocarbons.
Common Reagents and Conditions:
Oxidation: KMnO4 in an aqueous medium at room temperature.
Reduction: Hydrogen gas with Pd/C catalyst at elevated temperatures.
Substitution: Halogenation using bromine (Br2) in the presence of a Lewis acid catalyst.
Major Products:
Oxidation: Naphthalenone derivatives.
Reduction: Saturated hydrocarbons.
Substitution: Halogenated naphthalenone derivatives.
Scientific Research Applications
2-DECALONE has a wide range of applications in scientific research:
Chemistry: It is used as an intermediate in the synthesis of various organic compounds and as a model compound in studying hydrogenation reactions.
Biology: Its derivatives are investigated for potential biological activities, including antimicrobial and anticancer properties.
Medicine: Research is ongoing to explore its potential as a precursor for pharmaceutical compounds.
Industry: It is used in the production of fragrances, flavors, and other fine chemicals.
Mechanism of Action
The mechanism of action of 2-DECALONE involves its interaction with specific molecular targets. In biological systems, it may interact with enzymes and receptors, leading to various biochemical effects. The exact pathways and molecular targets are still under investigation, but it is believed to modulate cellular processes through its structural analogs .
Comparison with Similar Compounds
- 1,2,4-Metheno-1H-indene, octahydro-1,7a-dimethyl-5-(1-methylethyl)-
- Octahydro-1H-indole-2-carboxylic acid
Comparison: 2-DECALONE is unique due to its fully hydrogenated naphthalene ring system, which imparts distinct chemical properties compared to partially hydrogenated or non-hydrogenated analogs. Its stability and reactivity profile make it suitable for specific applications where other similar compounds may not be as effective .
Properties
IUPAC Name |
3,4,4a,5,6,7,8,8a-octahydro-1H-naphthalen-2-one | |
---|---|---|
Source | PubChem | |
URL | https://pubchem.ncbi.nlm.nih.gov | |
Description | Data deposited in or computed by PubChem | |
InChI |
InChI=1S/C10H16O/c11-10-6-5-8-3-1-2-4-9(8)7-10/h8-9H,1-7H2 | |
Source | PubChem | |
URL | https://pubchem.ncbi.nlm.nih.gov | |
Description | Data deposited in or computed by PubChem | |
InChI Key |
LGVJRKCQQHOWAU-UHFFFAOYSA-N | |
Source | PubChem | |
URL | https://pubchem.ncbi.nlm.nih.gov | |
Description | Data deposited in or computed by PubChem | |
Canonical SMILES |
C1CCC2CC(=O)CCC2C1 | |
Source | PubChem | |
URL | https://pubchem.ncbi.nlm.nih.gov | |
Description | Data deposited in or computed by PubChem | |
Molecular Formula |
C10H16O | |
Source | PubChem | |
URL | https://pubchem.ncbi.nlm.nih.gov | |
Description | Data deposited in or computed by PubChem | |
DSSTOX Substance ID |
DTXSID00862701 | |
Record name | 2-Decalone | |
Source | EPA DSSTox | |
URL | https://comptox.epa.gov/dashboard/DTXSID00862701 | |
Description | DSSTox provides a high quality public chemistry resource for supporting improved predictive toxicology. | |
Molecular Weight |
152.23 g/mol | |
Source | PubChem | |
URL | https://pubchem.ncbi.nlm.nih.gov | |
Description | Data deposited in or computed by PubChem | |
CAS No. |
4832-17-1 | |
Record name | 2-Decalone | |
Source | CAS Common Chemistry | |
URL | https://commonchemistry.cas.org/detail?cas_rn=4832-17-1 | |
Description | CAS Common Chemistry is an open community resource for accessing chemical information. Nearly 500,000 chemical substances from CAS REGISTRY cover areas of community interest, including common and frequently regulated chemicals, and those relevant to high school and undergraduate chemistry classes. This chemical information, curated by our expert scientists, is provided in alignment with our mission as a division of the American Chemical Society. | |
Explanation | The data from CAS Common Chemistry is provided under a CC-BY-NC 4.0 license, unless otherwise stated. | |
Record name | 2-Decalone | |
Source | ChemIDplus | |
URL | https://pubchem.ncbi.nlm.nih.gov/substance/?source=chemidplus&sourceid=0004832171 | |
Description | ChemIDplus is a free, web search system that provides access to the structure and nomenclature authority files used for the identification of chemical substances cited in National Library of Medicine (NLM) databases, including the TOXNET system. | |
Record name | 4832-17-1 | |
Source | DTP/NCI | |
URL | https://dtp.cancer.gov/dtpstandard/servlet/dwindex?searchtype=NSC&outputformat=html&searchlist=289484 | |
Description | The NCI Development Therapeutics Program (DTP) provides services and resources to the academic and private-sector research communities worldwide to facilitate the discovery and development of new cancer therapeutic agents. | |
Explanation | Unless otherwise indicated, all text within NCI products is free of copyright and may be reused without our permission. Credit the National Cancer Institute as the source. | |
Record name | 2-Decalone | |
Source | EPA DSSTox | |
URL | https://comptox.epa.gov/dashboard/DTXSID00862701 | |
Description | DSSTox provides a high quality public chemistry resource for supporting improved predictive toxicology. | |
Record name | Bicyclo[4.4.0]decan-2-one | |
Source | European Chemicals Agency (ECHA) | |
URL | https://echa.europa.eu/substance-information/-/substanceinfo/100.023.101 | |
Description | The European Chemicals Agency (ECHA) is an agency of the European Union which is the driving force among regulatory authorities in implementing the EU's groundbreaking chemicals legislation for the benefit of human health and the environment as well as for innovation and competitiveness. | |
Explanation | Use of the information, documents and data from the ECHA website is subject to the terms and conditions of this Legal Notice, and subject to other binding limitations provided for under applicable law, the information, documents and data made available on the ECHA website may be reproduced, distributed and/or used, totally or in part, for non-commercial purposes provided that ECHA is acknowledged as the source: "Source: European Chemicals Agency, http://echa.europa.eu/". Such acknowledgement must be included in each copy of the material. ECHA permits and encourages organisations and individuals to create links to the ECHA website under the following cumulative conditions: Links can only be made to webpages that provide a link to the Legal Notice page. | |
Retrosynthesis Analysis
AI-Powered Synthesis Planning: Our tool employs the Template_relevance Pistachio, Template_relevance Bkms_metabolic, Template_relevance Pistachio_ringbreaker, Template_relevance Reaxys, Template_relevance Reaxys_biocatalysis model, leveraging a vast database of chemical reactions to predict feasible synthetic routes.
One-Step Synthesis Focus: Specifically designed for one-step synthesis, it provides concise and direct routes for your target compounds, streamlining the synthesis process.
Accurate Predictions: Utilizing the extensive PISTACHIO, BKMS_METABOLIC, PISTACHIO_RINGBREAKER, REAXYS, REAXYS_BIOCATALYSIS database, our tool offers high-accuracy predictions, reflecting the latest in chemical research and data.
Strategy Settings
Precursor scoring | Relevance Heuristic |
---|---|
Min. plausibility | 0.01 |
Model | Template_relevance |
Template Set | Pistachio/Bkms_metabolic/Pistachio_ringbreaker/Reaxys/Reaxys_biocatalysis |
Top-N result to add to graph | 6 |
Feasible Synthetic Routes
Q1: What is the molecular formula and weight of 2-decalone?
A1: The molecular formula of this compound is C10H16O, and its molecular weight is 152.23 g/mol.
Q2: What are the key structural features of this compound?
A2: this compound is a bicyclic ketone composed of two fused cyclohexane rings with a ketone functional group at the 2-position. It exists as cis and trans isomers, differing in the relative orientation of the hydrogen atoms at the ring junction.
Q3: What spectroscopic techniques are used to characterize this compound?
A3: Researchers commonly employ techniques like Nuclear Magnetic Resonance (NMR) spectroscopy and Mass Spectrometry (MS) to characterize this compound. NMR helps determine the structure and stereochemistry, while MS provides information about the fragmentation pattern, useful for identifying the compound and its derivatives. [, ]
Q4: How does the stereochemistry of this compound influence its reactivity?
A4: The cis and trans isomers of this compound exhibit distinct reactivity profiles. For instance, in nucleophilic addition reactions, the trans isomer generally shows higher reactivity than the cis isomer. This difference arises from conformational factors, with the trans isomer favoring equatorial attack due to less steric hindrance. []
Q5: What are some important reactions involving this compound?
A5: this compound participates in various reactions, including: - Nucleophilic additions: Reactions with nucleophiles like hydroxylamine can occur at the carbonyl group, leading to the formation of the corresponding oximes. [] - Reductions: this compound can be reduced to the corresponding alcohols (decalols) using reducing agents such as sodium borohydride (NaBH4) or lithium aluminum hydride (LiAlH4). [, ] - Alkylations: The enolate of this compound can be generated and reacted with electrophiles to introduce alkyl substituents at the alpha-position. [, ] - Ring contractions: Treatment with reagents like thallium trinitrate (TTN) can lead to ring contraction, forming substituted cyclopentane derivatives. []
Q6: How is this compound used in organic synthesis?
A6: this compound serves as a versatile building block in organic synthesis. Researchers utilize it to construct more complex molecules, such as: - Terpenoids: The decalone framework is present in various natural products, including terpenoids. Synthetic strategies often utilize this compound derivatives to access these structurally diverse compounds. [, , ] - Polycyclic compounds: this compound can undergo transformations leading to the formation of polycyclic structures, which are important motifs in natural products and pharmaceutical compounds. [, ]
Q7: What is known about the metabolism of decalin, the parent hydrocarbon of this compound?
A7: Studies on rats show that decalin undergoes oxidation to form decalols and decalones. Notably, male rats exhibited greater susceptibility to kidney damage from decalin exposure, with the formation of this compound in the kidney extracts. This suggests a potential link between this compound and renal toxicity in male rats. [, ]
Q8: How do the metabolites of decalin contribute to its toxicity?
A8: The formation of decalones, particularly this compound, appears to play a role in decalin-induced nephrotoxicity in male rats. Research indicates that this compound might contribute to the accumulation of alpha2u-globulin in the kidneys, leading to hyaline droplet formation and ultimately renal damage. [, ]
Q9: Are there any known therapeutic applications of this compound or its derivatives?
A9: While no direct therapeutic applications of this compound are currently known, research exploring its derivatives and their biological activities is ongoing. For instance, some studies suggest potential antimicrobial properties of certain this compound derivatives. []
Q10: How is computational chemistry used in this compound research?
A10: Computational methods like molecular mechanics and ab initio calculations are employed to study the conformational behavior of this compound and its derivatives. These techniques provide valuable insights into the preferred conformations, energy barriers between conformers, and factors influencing reactivity. [, , ]
Q11: What is the significance of Structure-Activity Relationship (SAR) studies in this compound research?
A11: SAR studies are crucial for understanding how structural modifications to the this compound framework affect its biological activity. By systematically altering substituents and stereochemistry, researchers can identify key structural features responsible for desired activities and optimize compounds for specific applications. []
Q12: What is the environmental impact of this compound?
A12: Limited data is available on the environmental fate and ecotoxicological effects of this compound. As a bicyclic ketone, it may pose risks to aquatic life and potentially persist in the environment. Further research is needed to assess its biodegradability and develop strategies for its safe disposal and remediation. [, ]
Disclaimer and Information on In-Vitro Research Products
Please be aware that all articles and product information presented on BenchChem are intended solely for informational purposes. The products available for purchase on BenchChem are specifically designed for in-vitro studies, which are conducted outside of living organisms. In-vitro studies, derived from the Latin term "in glass," involve experiments performed in controlled laboratory settings using cells or tissues. It is important to note that these products are not categorized as medicines or drugs, and they have not received approval from the FDA for the prevention, treatment, or cure of any medical condition, ailment, or disease. We must emphasize that any form of bodily introduction of these products into humans or animals is strictly prohibited by law. It is essential to adhere to these guidelines to ensure compliance with legal and ethical standards in research and experimentation.