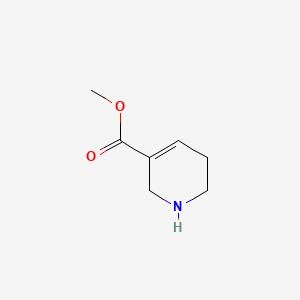
Guvacoline
Overview
Description
Guvacoline is a naturally occurring alkaloid that has been found in plant species such as Peganum harmala and Areca catechu. It has been studied extensively for its potential therapeutic applications due to its ability to interact with the nervous system. In
Scientific Research Applications
Quantitative Analysis of Alkaloids
Guvacoline, alongside other alkaloids like arecoline and arecaidine, has been studied for its presence and quantification in traditional Chinese medicine, particularly in Pericarpium Arecae (PA). Using high-performance liquid chromatography (HPLC), researchers have established methods for accurate and reliable detection of these alkaloids, which is crucial for quality control and content determination in PA (Yu et al., 2016).
In Vivo Presence in Saliva
Studies have shown that this compound, a nootropic agent, can be detected in vivo in the saliva of betel-quid chewers. This discovery was made using liquid-chromatography ion trap mass spectrometry, demonstrating this compound's presence in abundance in the aqueous extract with added lime (Kadi et al., 2013).
Analysis in Hair Samples
This compound has also been analyzed in the hair samples of betel nut abusers, using advanced techniques like ultra-high-performance liquid chromatography coupled to tandem mass spectrometry. This method helps document long-term exposure to this compound, providing insights into the dietary habits of certain populations (Gheddar et al., 2019).
Potential Action on Schizophrenia Symptoms
Research has suggested that this compound, as part of the betel alkaloids, might have a potential action on the symptoms of schizophrenia. A review of clinical literature data has indicated that high consumption of betel (which contains this compound) could be associated with lower positive symptoms in schizophrenia (Coppola & Mondola, 2012).
Cytotoxic and Genotoxic Effects Study
Although this study involves aspects outside the specified exclusion criteria (drug side effects), it's notable that research has been conducted on the cytotoxic and genotoxic effects of this compound and other areca nut-related compounds on human buccal epithelial cells. This research helps in understanding the pathobiological effects linked with betel quid chewing, which is relevant to oral cancer development (Sundqvist et al., 1989).
properties
IUPAC Name |
methyl 1,2,3,6-tetrahydropyridine-5-carboxylate | |
---|---|---|
Source | PubChem | |
URL | https://pubchem.ncbi.nlm.nih.gov | |
Description | Data deposited in or computed by PubChem | |
InChI |
InChI=1S/C7H11NO2/c1-10-7(9)6-3-2-4-8-5-6/h3,8H,2,4-5H2,1H3 | |
Source | PubChem | |
URL | https://pubchem.ncbi.nlm.nih.gov | |
Description | Data deposited in or computed by PubChem | |
InChI Key |
DYPLDWLIOGXSSE-UHFFFAOYSA-N | |
Source | PubChem | |
URL | https://pubchem.ncbi.nlm.nih.gov | |
Description | Data deposited in or computed by PubChem | |
Canonical SMILES |
COC(=O)C1=CCCNC1 | |
Source | PubChem | |
URL | https://pubchem.ncbi.nlm.nih.gov | |
Description | Data deposited in or computed by PubChem | |
Molecular Formula |
C7H11NO2 | |
Source | PubChem | |
URL | https://pubchem.ncbi.nlm.nih.gov | |
Description | Data deposited in or computed by PubChem | |
DSSTOX Substance ID |
DTXSID20197805 | |
Record name | Norarecoline | |
Source | EPA DSSTox | |
URL | https://comptox.epa.gov/dashboard/DTXSID20197805 | |
Description | DSSTox provides a high quality public chemistry resource for supporting improved predictive toxicology. | |
Molecular Weight |
141.17 g/mol | |
Source | PubChem | |
URL | https://pubchem.ncbi.nlm.nih.gov | |
Description | Data deposited in or computed by PubChem | |
CAS RN |
495-19-2 | |
Record name | Guvacoline | |
Source | CAS Common Chemistry | |
URL | https://commonchemistry.cas.org/detail?cas_rn=495-19-2 | |
Description | CAS Common Chemistry is an open community resource for accessing chemical information. Nearly 500,000 chemical substances from CAS REGISTRY cover areas of community interest, including common and frequently regulated chemicals, and those relevant to high school and undergraduate chemistry classes. This chemical information, curated by our expert scientists, is provided in alignment with our mission as a division of the American Chemical Society. | |
Explanation | The data from CAS Common Chemistry is provided under a CC-BY-NC 4.0 license, unless otherwise stated. | |
Record name | Norarecoline | |
Source | ChemIDplus | |
URL | https://pubchem.ncbi.nlm.nih.gov/substance/?source=chemidplus&sourceid=0000495192 | |
Description | ChemIDplus is a free, web search system that provides access to the structure and nomenclature authority files used for the identification of chemical substances cited in National Library of Medicine (NLM) databases, including the TOXNET system. | |
Record name | Norarecoline | |
Source | EPA DSSTox | |
URL | https://comptox.epa.gov/dashboard/DTXSID20197805 | |
Description | DSSTox provides a high quality public chemistry resource for supporting improved predictive toxicology. | |
Record name | GUVACOLINE | |
Source | FDA Global Substance Registration System (GSRS) | |
URL | https://gsrs.ncats.nih.gov/ginas/app/beta/substances/YT3OF85P98 | |
Description | The FDA Global Substance Registration System (GSRS) enables the efficient and accurate exchange of information on what substances are in regulated products. Instead of relying on names, which vary across regulatory domains, countries, and regions, the GSRS knowledge base makes it possible for substances to be defined by standardized, scientific descriptions. | |
Explanation | Unless otherwise noted, the contents of the FDA website (www.fda.gov), both text and graphics, are not copyrighted. They are in the public domain and may be republished, reprinted and otherwise used freely by anyone without the need to obtain permission from FDA. Credit to the U.S. Food and Drug Administration as the source is appreciated but not required. | |
Synthesis routes and methods
Procedure details
Retrosynthesis Analysis
AI-Powered Synthesis Planning: Our tool employs the Template_relevance Pistachio, Template_relevance Bkms_metabolic, Template_relevance Pistachio_ringbreaker, Template_relevance Reaxys, Template_relevance Reaxys_biocatalysis model, leveraging a vast database of chemical reactions to predict feasible synthetic routes.
One-Step Synthesis Focus: Specifically designed for one-step synthesis, it provides concise and direct routes for your target compounds, streamlining the synthesis process.
Accurate Predictions: Utilizing the extensive PISTACHIO, BKMS_METABOLIC, PISTACHIO_RINGBREAKER, REAXYS, REAXYS_BIOCATALYSIS database, our tool offers high-accuracy predictions, reflecting the latest in chemical research and data.
Strategy Settings
Precursor scoring | Relevance Heuristic |
---|---|
Min. plausibility | 0.01 |
Model | Template_relevance |
Template Set | Pistachio/Bkms_metabolic/Pistachio_ringbreaker/Reaxys/Reaxys_biocatalysis |
Top-N result to add to graph | 6 |
Feasible Synthetic Routes
Disclaimer and Information on In-Vitro Research Products
Please be aware that all articles and product information presented on BenchChem are intended solely for informational purposes. The products available for purchase on BenchChem are specifically designed for in-vitro studies, which are conducted outside of living organisms. In-vitro studies, derived from the Latin term "in glass," involve experiments performed in controlled laboratory settings using cells or tissues. It is important to note that these products are not categorized as medicines or drugs, and they have not received approval from the FDA for the prevention, treatment, or cure of any medical condition, ailment, or disease. We must emphasize that any form of bodily introduction of these products into humans or animals is strictly prohibited by law. It is essential to adhere to these guidelines to ensure compliance with legal and ethical standards in research and experimentation.