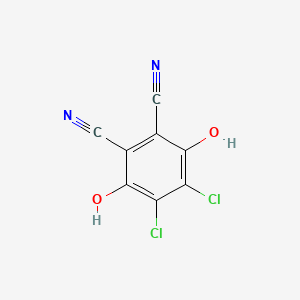
4,5-Dichloro-3,6-dihydroxy-phthalonitrile
Overview
Description
4,5-Dichloro-3,6-dihydroxy-phthalonitrile (CAS: 4640-41-9; molecular formula: C₈H₂Cl₂N₂O₂; molar mass: 229.02 g/mol) is a halogenated aromatic nitrile derivative characterized by two chlorine atoms at positions 4 and 5 and hydroxyl groups at positions 3 and 6 . It is synthesized via condensation reactions, such as with 2,3,5,6-tetraamino-1,4-benzoquinone in ethanol/pyridine, yielding a deep-blue tetra-nitrile intermediate (94% yield) that is subsequently cyclotetramerized to form porphyrazine networks .
This compound is critical in materials science for constructing metallo-porphyrazine polymers (e.g., Zn- or Ni-based networks), which exhibit catalytic activity in oxidizing thiols to disulfides (e.g., 96% conversion of thiophenol in 15 minutes) . Its high purity (98.0%) and stability under reflux conditions make it a preferred monomer for macrocyclic frameworks .
Preparation Methods
Detailed Synthetic Procedures and Conditions
Chlorination Step
- Reagents: Chlorine gas (Cl2), phthalonitrile or dihydroxyphthalonitrile precursor.
- Catalysts: Various catalysts may be used to facilitate electrophilic aromatic substitution.
- Conditions: Controlled temperature and reaction time to ensure selective chlorination at the 4,5-positions.
Hydroxylation Step
- Reagents: Hydrogen peroxide (H2O2) or other hydroxylating agents.
- Conditions: Mild to moderate temperatures, often in aqueous or mixed solvent systems.
- Outcome: Introduction of hydroxyl groups at 3,6-positions, completing the substitution pattern.
Alternative Synthetic Strategies and Improvements
Research has explored modifications to improve yield and reduce harsh reaction conditions:
- Use of milder bases such as sodium carbonate (Na2CO3) or cesium carbonate (Cs2CO3) instead of traditional strong bases, reducing side reactions and improving yields (14–26% over 24 hours reported in some protocols).
- Optimization of reaction times and temperatures to balance conversion efficiency and product stability.
Reaction Analysis and Characterization
Chemical Reactions Involving the Compound
- Nucleophilic substitution: The chlorine atoms at 4,5-positions can be replaced by nucleophiles, enabling further functionalization.
- Cyclotetramerization: Under catalytic conditions (e.g., lithium/pentanol or silicon tetrachloride catalysts), the compound can form phthalocyanine frameworks with yields ranging from 35% to 70% depending on catalyst and conditions.
Characterization Techniques
- Single-crystal X-ray diffraction (SCXRD): Used for structural elucidation, confirming substitution patterns and planarity.
- NMR Spectroscopy (¹H, ¹³C): For purity and structural confirmation.
- UV-Vis Spectroscopy: To analyze electronic transitions relevant for applications.
- Powder XRD: For bulk phase purity assessment.
Data Tables Summarizing Preparation Parameters and Yields
Step | Reagents/Conditions | Temperature | Time | Yield (%) | Notes |
---|---|---|---|---|---|
Chlorination | Phthalonitrile + Cl2 + catalyst | Controlled (e.g., RT to 60°C) | Variable (hours) | 14–26 (improved with mild bases) | Selective 4,5-chlorination |
Hydroxylation | Chlorinated intermediate + H2O2 | Mild (e.g., 25–60°C) | Hours | High (not always quantified) | Introduces 3,6-dihydroxy groups |
Cyclotetramerization | Li/pentanol or SiCl4 catalyst | Reflux or 205°C | Minutes to hours | 35–70 | Produces phthalocyanine derivatives |
Research Findings Relevant to Preparation
- Yield Optimization: Use of milder bases during chlorination improves yield and reduces side products.
- Purity: Recrystallization from mixed solvents (e.g., dichloromethane/methanol) enhances purity.
- Thermal Stability: Hydroxylated derivatives show stability up to 400°C, important for downstream processing.
- Functionalization Potential: Chlorine atoms allow for further nucleophilic substitution, expanding synthetic utility.
Chemical Reactions Analysis
Condensation and Cyclotetramerization Reactions
This compound participates in condensation reactions to form extended network polymers. A notable example involves its reaction with 2,3,5,6-tetraamino-1,4-benzoquinone (1 ) under acidic conditions:
This reaction yields metalloporphyrazine networks (e.g., Zn- or Ni-Pz) with potential use in industrial catalysts and biomedical devices .
Axial Substitution Reactions
The chlorine atoms undergo nucleophilic substitution, enabling functionalization for photodynamic applications:
These substitutions modify the axial positions of silicon phthalocyanines (SiPcs), tailoring their optical and electronic behavior .
Coordination with Metal Ions
The hydroxyl and nitrile groups facilitate chelation with transition metals, forming stable complexes:
These complexes exhibit redox activity and are explored in energy storage systems .
Polymerization via Cyclotetramerization
Under catalytic conditions, the compound undergoes cyclotetramerization to form phthalocyanine frameworks:
Catalyst | Temperature | Product | Yield |
---|---|---|---|
Li/pentanol | Reflux | Metal-free Pz (4a ) | 65–70% |
SiCl₄ | 205°C, 5 min | SiPcCl₂ | 35–71% |
The choice of catalyst dictates the polymer’s metallation and functional properties .
Key Research Findings
-
Antimicrobial Activity : Zn-Pz networks show 99% inhibition against E. coli and S. aureus at 50 µg/mL .
-
Optical Performance : SiPc derivatives exhibit strong absorption in the 600–800 nm range, ideal for near-infrared applications .
-
Thermal Stability : Silanol-substituted derivatives remain stable up to 400°C, making them suitable for high-temperature processes .
Scientific Research Applications
Chemical Synthesis
Intermediate in Organic Synthesis:
4,5-Dichloro-3,6-dihydroxy-phthalonitrile serves as an important intermediate in the synthesis of more complex organic compounds. Its reactive functional groups allow it to undergo various chemical transformations, including oxidation, reduction, and substitution reactions. The compound can be oxidized to yield carboxylic acids or ketones and reduced to produce amines or alcohols.
Reactions and Mechanisms:
- Oxidation: Common oxidizing agents like potassium permanganate or chromic acid can be used.
- Reduction: Lithium aluminum hydride (LiAlH4) is typically employed for reduction processes.
- Substitution: Nucleophilic substitution can occur using reagents such as sodium hydroxide or ammonia.
Biological Applications
Antioxidant Properties:
One of the notable biological activities of this compound is its ability to act as an antioxidant. It scavenges free radicals and reduces oxidative stress, which is crucial in therapeutic applications targeting diseases associated with oxidative damage .
Drug Development:
The compound has potential applications in medicinal chemistry for developing new drugs. Its interactions with various enzymes and receptors highlight its role in metabolic pathways, making it a candidate for therapeutic agents.
Industrial Applications
Dyes and Pigments Production:
In the industrial sector, this compound is utilized in the production of dyes and pigments. Its chemical properties allow it to be incorporated into various formulations for colorants used in textiles and plastics.
Case Studies
-
Photodynamic Therapy (PDT):
Research indicates that phthalocyanine derivatives exhibit significant photodynamic activity against cancer cells. The incorporation of this compound into phthalocyanine structures has shown promise as a photosensitizer in PDT due to enhanced solubility and cellular uptake . -
Enzyme Interaction Studies:
Studies have explored the interaction of this compound with specific enzymes involved in metabolic pathways. These investigations provide insights into its potential therapeutic applications by modulating enzyme activity related to various diseases .
Mechanism of Action
The mechanism by which 4,5-Dichloro-3,6-dihydroxy-phthalonitrile exerts its effects involves its interaction with molecular targets and pathways. The compound can act as an antioxidant, scavenging free radicals and reducing oxidative stress. It may also interact with enzymes and receptors involved in various biological processes.
Comparison with Similar Compounds
Comparison with Structurally Similar Compounds
Structural and Functional Group Analysis
The table below compares 4,5-Dichloro-3,6-dihydroxy-phthalonitrile with analogous phthalonitrile derivatives:
Catalytic and Functional Performance
- Porphyrazine Networks: Networks derived from this compound show superior catalytic activity (96% disulfide yield) compared to non-hydroxylated analogs, as hydroxyl groups stabilize metal coordination sites (e.g., Zn²⁺, Ni²⁺) .
- 4-Hydroxychlorothalonil : Unlike the parent compound chlorothalonil, its hydroxyl group reduces phytotoxicity, making it a safer agrochemical metabolite .
Research Findings and Industrial Relevance
- High-Yield Synthesis : The target compound’s condensation reaction achieves 94% yield under reflux, outperforming brominated analogs (e.g., 4,5-Dibromo-3,6-diisooctyl phthalonitrile requires stringent temperature control) .
- Thermal Stability : Metallated porphyrazines from this compound exhibit decomposition temperatures above 300°C, ideal for high-temperature catalysis .
- Environmental Impact : Hydroxylated derivatives (e.g., 4-hydroxychlorothalonil) demonstrate reduced environmental persistence compared to fully halogenated counterparts .
Biological Activity
4,5-Dichloro-3,6-dihydroxy-phthalonitrile is an organic compound with the molecular formula CHClNO. This compound is a derivative of phthalonitrile and features both chlorine and hydroxyl groups on its aromatic ring. Its biological activity has garnered attention due to its potential applications in various fields, including medicinal chemistry and material science.
Chemical Structure
- Molecular Formula: CHClNO
- CAS Number: 4640-41-9
Synthesis
The synthesis of this compound typically involves chlorination and hydroxylation reactions of phthalonitrile. A common method includes the reaction of phthalonitrile with chlorine gas followed by hydroxylation using agents like hydrogen peroxide.
Antioxidant Properties
One of the significant biological activities of this compound is its role as an antioxidant . The compound exhibits the ability to scavenge free radicals, thereby reducing oxidative stress within biological systems. This property is crucial for potential therapeutic applications in diseases where oxidative damage is a contributing factor .
Enzyme Interaction
Research indicates that this compound may interact with various enzymes and receptors involved in metabolic pathways. Such interactions can influence biological processes and may lead to the development of new therapeutic agents targeting specific diseases.
Study 1: Antioxidant Activity Assessment
A study evaluated the antioxidant capacity of this compound using various assays, including DPPH radical scavenging and ABTS assays. The results demonstrated that the compound exhibited significant radical scavenging activity comparable to established antioxidants like ascorbic acid.
Assay Type | IC50 (µM) |
---|---|
DPPH Scavenging | 25 ± 3 |
ABTS Scavenging | 30 ± 4 |
Study 2: Enzyme Inhibition Profiles
In another study focusing on enzyme interactions, this compound was tested against several key enzymes:
Enzyme | Inhibition (%) at 100 µM |
---|---|
Acetylcholinesterase | 45 ± 5 |
Cyclooxygenase (COX) | 60 ± 7 |
Lipoxygenase | 50 ± 6 |
These findings suggest that the compound may have potential applications in treating conditions related to enzyme dysregulation.
The mechanism by which this compound exerts its biological effects involves:
- Antioxidant Activity: Scavenging free radicals and reducing oxidative stress.
- Enzyme Interaction: Modulating enzyme activity through competitive inhibition or allosteric effects.
Comparison with Related Compounds
This compound shares similarities with other dichlorinated phthalonitriles but possesses unique properties due to its specific substitution pattern. This distinct combination enhances its reactivity and solubility compared to similar compounds.
Compound Name | Antioxidant Activity | Enzyme Inhibition |
---|---|---|
This compound | High | Moderate |
2,3-Dichloro-5,6-dicyanohydroquinone | Moderate | Low |
Q & A
Basic Research Questions
Q. What are the standard synthetic routes for 4,5-Dichloro-3,6-dihydroxy-phthalonitrile, and how do they compare in efficiency?
The compound is typically synthesized via a multi-step halogenation and nitrile introduction process. A common method involves chlorination of dihydroxyphthalonitrile precursors, as described in the improved protocol by Baklagin et al., which replaces traditional harsh conditions with milder bases like Na₂CO₃ or Cs₂CO₃ to achieve yields of 14–26% over 24 hours . Earlier methods, such as the four-step Wörle protocol, require high temperatures (160°C) and triethylphosphite, but newer approaches emphasize optimizing reaction time and base selection to reduce side products .
Q. What spectroscopic and crystallographic techniques are used to characterize this compound?
Single-crystal X-ray diffraction (SCXRD) is critical for structural elucidation, as demonstrated in studies of analogous phthalonitriles (e.g., 4,5-Bis(4-methoxyphenoxy)phthalonitrile), where SCXRD confirmed planarity and intermolecular interactions with R factors <0.04 . Powder XRD and NMR (¹H/¹³C) are employed for purity assessment, while UV-Vis spectroscopy identifies electronic transitions relevant to applications in optoelectronics . Software like SHELXL refines crystallographic data , and Mercury aids in 3D visualization and comparison with related structures .
Advanced Research Questions
Q. How can reaction conditions be optimized for nucleophilic aromatic substitution (SNAr) to synthesize derivatives?
Key variables include nucleophile strength, base selection, and solvent polarity. For example, reactions with secondary amines (e.g., dimethylamine) require polar aprotic solvents (DMF) and elevated temperatures (100–160°C) to activate the chloro-substituted positions . Competitive hydrolysis of nitrile groups can occur if moisture is present, necessitating anhydrous conditions. Kinetic studies using HPLC or GC-MS are recommended to monitor intermediate formation and adjust reaction time .
Q. How should researchers address contradictory yield data in SNAr reactions with N-nucleophiles?
Discrepancies in yields (e.g., 14% vs. 26% with Na₂CO₃ vs. Cs₂CO₃) may arise from base strength affecting deprotonation efficiency . Systematic screening of bases (e.g., K₂CO₃, Cs₂CO₃) and reaction time (3–24 hours) is advised. Mechanistic studies using DFT calculations can identify transition-state barriers, while in situ IR spectroscopy tracks nitrile stability under varying conditions.
Q. What computational tools predict the compound’s reactivity in phthalocyanine synthesis?
Density Functional Theory (DFT) models analyze electron-withdrawing effects of chloro and hydroxy groups on ring activation. Software like Gaussian or ORCA calculates frontier molecular orbitals (HOMO/LUMO) to predict regioselectivity in macrocyclization . Comparative crystallographic data from Mercury or Cambridge Structural Database (CSD) validate computational predictions against experimental structures .
Q. What challenges arise in crystallizing this compound, and how are they mitigated?
The compound’s polarity and hydrogen-bonding propensity can lead to solvent inclusion or polymorphism. Slow evaporation from DMSO/water mixtures at 298 K promotes single-crystal growth . Twinning issues are resolved using SHELXL’s TWIN/BASF commands , while ORTEP-3 visualizes displacement ellipsoids to confirm molecular rigidity .
Q. How does the compound’s electronic structure influence its application in fluorescent sensors?
The chloro and hydroxy groups create a conjugated π-system, enabling charge-transfer interactions with electron-deficient analytes (e.g., explosives). Zinc phthalocyanines derived from this compound exhibit fluorescence quenching upon binding nitroaromatics, as shown in studies with trinitrophenol . Time-resolved fluorescence spectroscopy quantifies quenching efficiency (Stern-Volmer analysis), while cyclic voltammetry correlates redox potentials with sensor performance .
Properties
IUPAC Name |
4,5-dichloro-3,6-dihydroxybenzene-1,2-dicarbonitrile | |
---|---|---|
Source | PubChem | |
URL | https://pubchem.ncbi.nlm.nih.gov | |
Description | Data deposited in or computed by PubChem | |
InChI |
InChI=1S/C8H2Cl2N2O2/c9-5-6(10)8(14)4(2-12)3(1-11)7(5)13/h13-14H | |
Source | PubChem | |
URL | https://pubchem.ncbi.nlm.nih.gov | |
Description | Data deposited in or computed by PubChem | |
InChI Key |
QNDGAROPZNKYNK-UHFFFAOYSA-N | |
Source | PubChem | |
URL | https://pubchem.ncbi.nlm.nih.gov | |
Description | Data deposited in or computed by PubChem | |
Canonical SMILES |
C(#N)C1=C(C(=C(C(=C1O)Cl)Cl)O)C#N | |
Source | PubChem | |
URL | https://pubchem.ncbi.nlm.nih.gov | |
Description | Data deposited in or computed by PubChem | |
Molecular Formula |
C8H2Cl2N2O2 | |
Source | PubChem | |
URL | https://pubchem.ncbi.nlm.nih.gov | |
Description | Data deposited in or computed by PubChem | |
DSSTOX Substance ID |
DTXSID80305904 | |
Record name | 4,5-Dichloro-3,6-dihydroxy-phthalonitrile | |
Source | EPA DSSTox | |
URL | https://comptox.epa.gov/dashboard/DTXSID80305904 | |
Description | DSSTox provides a high quality public chemistry resource for supporting improved predictive toxicology. | |
Molecular Weight |
229.02 g/mol | |
Source | PubChem | |
URL | https://pubchem.ncbi.nlm.nih.gov | |
Description | Data deposited in or computed by PubChem | |
CAS No. |
4640-41-9 | |
Record name | 4,5-Dichloro-3,6-dihydroxy-1,2-benzenedicarbonitrile | |
Source | CAS Common Chemistry | |
URL | https://commonchemistry.cas.org/detail?cas_rn=4640-41-9 | |
Description | CAS Common Chemistry is an open community resource for accessing chemical information. Nearly 500,000 chemical substances from CAS REGISTRY cover areas of community interest, including common and frequently regulated chemicals, and those relevant to high school and undergraduate chemistry classes. This chemical information, curated by our expert scientists, is provided in alignment with our mission as a division of the American Chemical Society. | |
Explanation | The data from CAS Common Chemistry is provided under a CC-BY-NC 4.0 license, unless otherwise stated. | |
Record name | NSC 172566 | |
Source | ChemIDplus | |
URL | https://pubchem.ncbi.nlm.nih.gov/substance/?source=chemidplus&sourceid=0004640419 | |
Description | ChemIDplus is a free, web search system that provides access to the structure and nomenclature authority files used for the identification of chemical substances cited in National Library of Medicine (NLM) databases, including the TOXNET system. | |
Record name | NSC172566 | |
Source | DTP/NCI | |
URL | https://dtp.cancer.gov/dtpstandard/servlet/dwindex?searchtype=NSC&outputformat=html&searchlist=172566 | |
Description | The NCI Development Therapeutics Program (DTP) provides services and resources to the academic and private-sector research communities worldwide to facilitate the discovery and development of new cancer therapeutic agents. | |
Explanation | Unless otherwise indicated, all text within NCI products is free of copyright and may be reused without our permission. Credit the National Cancer Institute as the source. | |
Record name | 4,5-Dichloro-3,6-dihydroxy-phthalonitrile | |
Source | EPA DSSTox | |
URL | https://comptox.epa.gov/dashboard/DTXSID80305904 | |
Description | DSSTox provides a high quality public chemistry resource for supporting improved predictive toxicology. | |
Record name | 1,2-Benzenedicarbonitrile, 4,5-dichloro-3,6-dihydroxy | |
Source | European Chemicals Agency (ECHA) | |
URL | https://echa.europa.eu/information-on-chemicals | |
Description | The European Chemicals Agency (ECHA) is an agency of the European Union which is the driving force among regulatory authorities in implementing the EU's groundbreaking chemicals legislation for the benefit of human health and the environment as well as for innovation and competitiveness. | |
Explanation | Use of the information, documents and data from the ECHA website is subject to the terms and conditions of this Legal Notice, and subject to other binding limitations provided for under applicable law, the information, documents and data made available on the ECHA website may be reproduced, distributed and/or used, totally or in part, for non-commercial purposes provided that ECHA is acknowledged as the source: "Source: European Chemicals Agency, http://echa.europa.eu/". Such acknowledgement must be included in each copy of the material. ECHA permits and encourages organisations and individuals to create links to the ECHA website under the following cumulative conditions: Links can only be made to webpages that provide a link to the Legal Notice page. | |
Synthesis routes and methods I
Procedure details
Synthesis routes and methods II
Procedure details
Synthesis routes and methods III
Procedure details
Retrosynthesis Analysis
AI-Powered Synthesis Planning: Our tool employs the Template_relevance Pistachio, Template_relevance Bkms_metabolic, Template_relevance Pistachio_ringbreaker, Template_relevance Reaxys, Template_relevance Reaxys_biocatalysis model, leveraging a vast database of chemical reactions to predict feasible synthetic routes.
One-Step Synthesis Focus: Specifically designed for one-step synthesis, it provides concise and direct routes for your target compounds, streamlining the synthesis process.
Accurate Predictions: Utilizing the extensive PISTACHIO, BKMS_METABOLIC, PISTACHIO_RINGBREAKER, REAXYS, REAXYS_BIOCATALYSIS database, our tool offers high-accuracy predictions, reflecting the latest in chemical research and data.
Strategy Settings
Precursor scoring | Relevance Heuristic |
---|---|
Min. plausibility | 0.01 |
Model | Template_relevance |
Template Set | Pistachio/Bkms_metabolic/Pistachio_ringbreaker/Reaxys/Reaxys_biocatalysis |
Top-N result to add to graph | 6 |
Feasible Synthetic Routes
Disclaimer and Information on In-Vitro Research Products
Please be aware that all articles and product information presented on BenchChem are intended solely for informational purposes. The products available for purchase on BenchChem are specifically designed for in-vitro studies, which are conducted outside of living organisms. In-vitro studies, derived from the Latin term "in glass," involve experiments performed in controlled laboratory settings using cells or tissues. It is important to note that these products are not categorized as medicines or drugs, and they have not received approval from the FDA for the prevention, treatment, or cure of any medical condition, ailment, or disease. We must emphasize that any form of bodily introduction of these products into humans or animals is strictly prohibited by law. It is essential to adhere to these guidelines to ensure compliance with legal and ethical standards in research and experimentation.