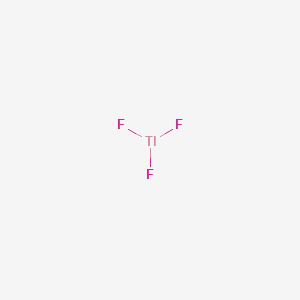
Thallium fluoride (TlF3)
- Click on QUICK INQUIRY to receive a quote from our team of experts.
- With the quality product at a COMPETITIVE price, you can focus more on your research.
Overview
Description
Thallium fluoride, also known as thallium trifluoride, is an inorganic compound with the chemical formula TlF3. It is a white solid that is primarily of theoretical interest. Thallium fluoride adopts the same structure as bismuth trifluoride, featuring eight-coordinate thallium (III) centers .
Preparation Methods
Thallium fluoride can be synthesized through various methods. One common synthetic route involves the reaction of thallium (III) oxide (Tl2O3) with hydrofluoric acid (HF). The reaction is typically carried out under controlled conditions to ensure the complete conversion of thallium (III) oxide to thallium fluoride. The reaction can be represented as follows:
Tl2O3+6HF→2TlF3+3H2O
Chemical Reactions Analysis
Thallium fluoride undergoes various chemical reactions, including oxidation, reduction, and substitution reactions. Some common reagents and conditions used in these reactions include:
Oxidation: Thallium fluoride can be oxidized to form thallium (III) oxide (Tl2O3) when exposed to strong oxidizing agents.
Reduction: Thallium fluoride can be reduced to thallium (I) fluoride (TlF) using reducing agents such as hydrogen gas (H2) or sodium borohydride (NaBH4).
Substitution: Thallium fluoride can undergo substitution reactions with other halides to form mixed halides, such as thallium bromofluoride (TlF2Br).
The major products formed from these reactions depend on the specific reagents and conditions used .
Scientific Research Applications
Thallium fluoride has several scientific research applications, particularly in the fields of chemistry and materials science. Some of its notable applications include:
Catalysis: Thallium fluoride is used as a catalyst in various chemical reactions, including organic synthesis and polymerization reactions.
Materials Science: Thallium fluoride is used in the production of specialized optical materials, such as infrared lenses and windows, due to its unique optical properties.
Analytical Chemistry: Thallium fluoride is used as a reagent in analytical chemistry for the detection and quantification of various elements and compounds.
Mechanism of Action
The mechanism of action of thallium fluoride involves its ability to interact with various molecular targets and pathways. Thallium fluoride can form complexes with other molecules, altering their chemical properties and reactivity. The specific molecular targets and pathways involved depend on the particular application and reaction conditions .
Comparison with Similar Compounds
Thallium fluoride can be compared with other similar compounds, such as aluminum fluoride (AlF3), gallium fluoride (GaF3), and indium fluoride (InF3). These compounds share similar chemical properties and structures, but each has unique characteristics that make them suitable for different applications. For example:
Aluminum Fluoride (AlF3): Used as a catalyst in the production of aluminum and in the manufacture of ceramics.
Gallium Fluoride (GaF3): Used in the production of semiconductors and as a catalyst in organic synthesis.
Indium Fluoride (InF3): Used in the production of optical materials and as a reagent in analytical chemistry
Thallium fluoride’s uniqueness lies in its specific coordination structure and its limited industrial applications compared to its counterparts.
Properties
CAS No. |
7783-57-5 |
---|---|
Molecular Formula |
F3Tl |
Molecular Weight |
261.379 g/mol |
IUPAC Name |
thallium(3+);trifluoride |
InChI |
InChI=1S/3FH.Tl/h3*1H;/q;;;+3/p-3 |
InChI Key |
BOUDEKXATYHWHY-UHFFFAOYSA-K |
SMILES |
F[Tl](F)F |
Canonical SMILES |
[F-].[F-].[F-].[Tl+3] |
Key on ui other cas no. |
7783-57-5 |
Origin of Product |
United States |
Disclaimer and Information on In-Vitro Research Products
Please be aware that all articles and product information presented on BenchChem are intended solely for informational purposes. The products available for purchase on BenchChem are specifically designed for in-vitro studies, which are conducted outside of living organisms. In-vitro studies, derived from the Latin term "in glass," involve experiments performed in controlled laboratory settings using cells or tissues. It is important to note that these products are not categorized as medicines or drugs, and they have not received approval from the FDA for the prevention, treatment, or cure of any medical condition, ailment, or disease. We must emphasize that any form of bodily introduction of these products into humans or animals is strictly prohibited by law. It is essential to adhere to these guidelines to ensure compliance with legal and ethical standards in research and experimentation.